
- •Materials and Methods
- •Apparatus and Reagents
- •Liver Decellularization
- •Histological Analysis
- •Immunostaining
- •Liver Scaffold Seeding
- •In Vivo Implantation of Scaffolds
- •Statistical Analysis
- •Results
- •Decellularization of the Porcine Liver
- •Preservation of Liver Structures
- •Cytotoxicity of Decellularized Liver Matrix
- •Immunogenicity of Liver Matrix Scaffolds
- •Discussion
- •Acknowledgments
- •Supplemental Data
- •References

Decellularized Porcine Liver
Figure 1 Porcine liver decellularization. Whole native liver (A) before perfusion with Triton X-100 (B) and SDS (C). Indicated by panels on the SDS-treated liver, the preserved major (D) and minor (E) vessels are visible at the end of the protocol. Native liver sections stained with H&E (F) and Masson’s trichrome (G) help illustrate the complete cellular removal seen in their respective decellularized counterparts (H and I). Arrows indicate preserved vessels in decellularized matrices compared with native structures. Scale bar Z 100 mm (FeI).
detergents and, in some cases, enzymatic means, but the resultant matrices must demonstrate noncytotoxocity and, moreover, scaffolds must support cell growth to be considered for organ bioengineering purposes (Figure 3). HepG2 cells were statically seeded onto decellularized liver scaffolds and maintained for
Figure 2 Preservation of discreet ultrastructural components. Positive immunostaining for vascular elements elastin (ELN; A) and laminin (LN; B). C: Scanning electron micrographs of the decellularized porcine liver showed distinct areas of the capsule, vessels, and parenchyma, shown under higher magnification in DeF, respectively. Original magnification: 50 (C); 200 (DeF). Scale bar Z 200 mm (A and B).
Figure 3 In vitro noncytotoxicity of porcine liver matrix scaffolds. HepG2 cells were statically seeded onto scaffolds and cultured out to 21 days to determine whether any cytotoxic compounds would be released from the decellularized matrices. TUNEL staining of the scaffolds at 7 (A) and 21 (B) days indicated minimal apoptotic response of HepG2 cells to liver scaffolds, in both close contact with scaffolds and within cell masses (arrows), compared with DNase-treated positive control samples (C). LM, liver matrix. Scale bar Z 50 mm (AeC).
up to 21 days, when dense cell layers were observed. Cells at day 7 (Figure 3A) and day 21 (Figure 3B) did not exhibit apoptotic markers, as displayed in control samples with degraded DNA (Figure 3C) and confirmed by TUNEL staining. Cells were observed attached to the surfaces of matrices, with minimal penetration into the liver matrix scaffold.
Immunogenicity of Liver Matrix Scaffolds
To investigate the immunogenicity of our decellularized scaffolds (rodent and porcine), we implanted naked scaffolds (Figure 4, C and D) into the s.c. dorsal adipose tissue of rats (Figure 4A). Scaffolds were recovered (Figure 4B) with surrounding tissue intact to measure cellular infiltration and host response, with no visible fibrous encapsulation of scaffolds or exudates observed at implantation sites. After 7 days (Figure 4, E and F), cells had migrated into both allogeneic (rat) and xenogeneic (porcine) scaffolding, with no signs of an inflammatory response. Over 28 days (Figure 4, G and H), host cells continued to populate each recovered scaffold, with no noticeable adverse host response surrounding the matrices. Systemic white blood cell counts were collected from animals during the experiment and compared with rodents who underwent implantation surgeries, but received no matrix implant (sham). Total white blood cell counts (Figure 4I), lymphocyte counts (Figure 4J), and monocyte counts (Figure 4K) were not significantly different between groups (P > 0.05) at 2, 7, 14, and 28 days, indicating no major systemic host response compared with normal surgical recovery. Lymphocyte count results were supported by little to no CD3þ T-cell activation at the implantation site, as seen over the 28 days in either group (Figure 5, A and B). Many cells that infiltrated the implanted scaffolds were positive for the panmacrophage phenotypic marker, CD68 (Figure 5, C and D). However, as seen previously, neither the monocyte counts (as macrophage precursors) (Figure 4K) nor the cells expressing markers indicating adoption of the M1 (CD86þ) or M2 (CD206þ) phenotypes (Figure 5, EeH) showed an increase.
Discussion
In this study, we developed a successful protocol for the generation of clinically relevant sized decellularized porcine
The American Journal of Pathology - ajp.amjpathol.org |
561 |

Mirmalek-Sani et al
Figure 4 Host response. In all groups, grafts were implanted s.c. in the dorsal adipose tissue of a given animal (A) and harvested intact for histological analysis (B), with no visible encapsulation or exudate at the implantation site. Arrows denote the four scaffolds implanted per animal. C and D: H&E staining of nonimplanted biopsy specimens of decellularized allogeneic (Allo; rat) and xenogeneic (Xeno; pig) livers indicated no cellular material in the scaffolds before implantation. E and F: Day 7 (d7) allogeneic and xenogeneic explants indicated cellular infiltration of the scaffolding material, with no fibrous encapsulation under microscopic examination. G and H: Day 28 (d28) explants continued to indicate cellular activity inside both allogeneic and xenogeneic scaffolding materials, with no encapsulation of the scaffold. Systemic white blood cell analysis over the length of the experiment indicated no significant difference (P > 0.05) between implanted allograft or xenograft scaffolds compared with shameoperated on animals (no scaffold implantation) in total white blood cell count (I), lymphocyte count (J), or monocyte count (K), indicating no major host response to the ECM materials. Significantly decreased counts in all groups were observed over time (P < 0.05). Scale bar Z 100 mm (CeH).
livers, as derived from our previous rodent liver decellularization method.28 These studies demonstrated, after detergent perfusion and rinsing, cellular clearance and preservation of the vasculature tree and ECM proteins, as shown by our
previous scaffolds24,28 or scaffolds generated by other groups.26,30,42 Maintenance of patent blood vessel structures
provides an essential nutrient distribution network for whole organ regenerative studies. Without blood vessel structures, static transport limitations within the scaffolding material will generate necrotic pockets because cell proliferation would leave the cells most distant from nutrient sources starved.38 With blood vessel structures, we avoid such a
detrimental situation and instead replicate the body’s nutrient delivery and toxin removal by perfusing the vasculature at model pressure and flow conditions.
The vasculature also provides a lattice structure for endothelial cell attachment and proliferation that will be crucial for prevention of thrombosis on vascular anastomosis within the recipient. Specifically for the liver, an implantable unit will need to be directly connected to the host vasculature. A previous study demonstrated the successful decellularization and implantation25 of porcine kidney scaffolds, which con- firmed that an acellular matrix could withstand the strain of surgical implantation and exposure to arterial blood flow. Our
562 |
ajp.amjpathol.org - The American Journal of Pathology |
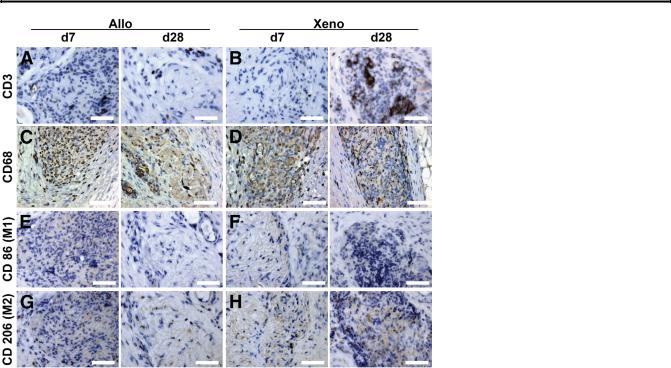
Decellularized Porcine Liver
Figure 5 Cell-specific activation. Anti-CD3þ staining of allogeneic (Allo; A) and xenogeneic (Xeno; B) liver explant tissues on early [day 7 (d7)] and late [day 28 (d28)] time points indicated minimal or no CD3þ T-cell activation. CD68þ pan-macrophages were seen within implanted allogeneic (C) and xenogeneic (D) matrices over the duration of the experiment. Staining for macrophage polarity indicated minimal or no activation of CD86þ (M1) in either allogeneic (E) or xenogeneic (F) scaffolds over early and late time points. G and H: Similar results were observed for the presence of CD206þ (M2) macrophages, with limited positive staining in xenogeneic samples. Scale bar Z 50 mm (AeH).
liver scaffolds have also maintained the integrity of the organ’s capsule, critical for preventing increases in permeability and circulation leaks that would be detrimental in maintaining the closed-loop vasculature.
The preservation of basement membrane proteins, such as collagens, fibronectin (data not shown), elastins, and laminins, has been shown to maintain hepatic cell function for longer time periods than classic substrates.43e45 As well as primary cells, embryonic and mesenchymal stem cells have been infused into decellularized scaffolds and shown to develop organ-specific phenotypes and functions simply through scaffold interaction.7,42 Our present studies focused on minimizing the cytotoxicity of our scaffold material and demonstrated no contact or soluble toxicity, with maintenance of HepG2 hepatoblastoma cells over 21 days. Our follow-up research is focused on the co-culture of primary hepatic and endothelial cells on the matrix material for the development of a functional and implantable liver device.
Related to cellular engraftment, viability, and proliferation, there are ongoing investigations into the effects of g sterilization and peracetic acid chemical sterilization on scaffold elasticity.46 It has been reported that the elastic modulus of the underlying substrate can directly affect cell attachment, behavior, and viability.47,48 Anecdotal observations suggested significant alteration of the physical scaffold
stiffness pre-g and post-g sterilization, supporting previous studies.49,50 However, specifics into the impact these changes
have on tissue regeneration are limited.8 We believe each step in our decellularization protocol should focus only on the removal of the donor cellular material, while minimizing the impact on inherent mechanical properties of the substratum. Ongoing studies are examining alternatives to irradiation to achieve whole organ sterilization before reseeding.
Before progressing to the transplantation of regenerated liver tissue, a basic understanding of the host immune response toward the scaffolding material is essential to determine whether a decellularized biomaterial is able to affect proin- flammatory macrophage activation.38,51 Our studies assessed the response to rodent and porcine decellularized liver biopsy specimens within a rodent model (allograft versus xenograft proteins). In doing so, we reaffirmed the similarity of the scaffold components between species and reduced future concerns over matrix properties when evaluating host immune response to a recellularized scaffold. The influx of macrophages without activation, as seen with our scaffold materials, is not uncommon and may, instead, be an indication of the influence of potential factors stored in the matrix material itself.32,35
In summary, our protocol adaptation was able to generate large-scale organ scaffolds of a clinically relevant size for further investigations of organ regeneration. Our matrices preserved essential ECM proteins for cell engraftment and function, as well as the vasculature required for nutrient distribution for whole organ reseeding. Neither our previously developed rodent scaffolds nor newly prepared porcine scaffolds elicited a host immune response, while also readily being colonized by host cells. These results indicate that a naturally derived ECM scaffold is viable for the next stages of regeneration of a bioengineered hepatic tissue.
Acknowledgments
We thank Dr. James Jordan and Magan Lane (Wake Forest University, Winston-Salem, NC) for the provision of pig livers and Daniel Deegan, Erica Wieser, and Kayla Trivette for technical support.
The American Journal of Pathology - ajp.amjpathol.org |
563 |

Mirmalek-Sani et al
Supplemental Data
Supplemental material for this article can be found at http://dx.doi.org/10.1016/j.ajpath.2013.05.002.
References
1.Organ Procurement and Transplantation Network (OPTN) and Scientific Registry of Transplant Recipients (SRTR). OPTN/SRTR 2011 Annual Data Report. Department of Health and Human Services, Health Resources and Services Administration, Healthcare Systems Bureau, Division of Transplantation; 2012. Available at http://srtr.transplant.hrsa.gov/annual_reports/2011/default.aspx.
2.Soto-Gutierrez A, Yagi H, Uygun BE, Navarro-Alvarez N, Uygun K, Kobayashi N, Yang YG, Yarmush ML: Cell delivery: from cell transplantation to organ engineering. Cell Transplant 2010, 19:655e665
3.Ekser B, Rigotti P, Gridelli B, Cooper DK: Xenotransplantation of solid organs in the pig-to-primate model. Transpl Immunol 2009, 21: 87e92
4.Badylak SF: Xenogeneic extracellular matrix as a scaffold for tissue reconstruction. Transpl Immunol 2004, 12:367e377
5.Baptista PM, Orlando G, Mirmalek-Sani SH, Siddiqui M, Atala A, Soker S: Whole organ decellularization: a tool for bioscaffold fabrication and organ bioengineering. Conf Proc IEEE Eng Med Biol Soc 2009, 2009:6526e6529
6.Orlando G, Baptista P, Birchall M, De Coppi P, Farney A, Guimar- aes-Souza NK, Opara E, Rogers J, Seliktar D, Shapira-Schweitzer K, Stratta RJ, Atala A, Wood KJ, Soker S: Regenerative medicine as applied to solid organ transplantation: current status and future challenges. Transpl Int 2011, 24:223e232
7.Ross EA, Williams MJ, Hamazaki T, Terada N, Clapp WL, Adin C, Ellison GW, Jorgensen M, Batich CD: Embryonic stem cells proliferate and differentiate when seeded into kidney scaffolds. J Am Soc Nephrol 2009, 20:2338e2347
8.Crapo PM, Gilbert TW, Badylak SF: An overview of tissue and whole organ decellularization processes. Biomaterials 2011, 32: 3233e3243
9.Ott HC, Matthiesen TS, Goh SK, Black LD, Kren SM, Netoff TI, Taylor DA: Perfusion-decellularized matrix: using nature’s platform to engineer a bioartificial heart. Nat Med 2008, 14:213e221
10.Wainwright JM, Czajka CA, Patel UB, Freytes DO, Tobita K, Gilbert TW, Badylak SF: Preparation of cardiac extracellular matrix from an intact porcine heart. Tissue Eng Part C-Me 2010, 16: 525e532
11.Ott HC, Clippinger B, Conrad C, Schuetz C, Pomerantseva I, Ikonomou L, Kotton D, Vacanti JP: Regeneration and orthotopic transplantation of a bioartificial lung. Nat Med 2010, 16:927e933
12.Petersen TH, Calle EA, Zhao L, Lee EJ, Gui L, Raredon MB, Gavrilov K, Yi T, Zhuang ZW, Breuer C, Herzog E, Niklason LE: Tissue-engineered lungs for in vivo implantation. Science 2010, 329:538e541
13.Song JJ, Kim SS, Liu ZL, Madsen JC, Mathisen DJ, Vacanti JP, Ott HC: Enhanced in vivo function of bioartificial lungs in rats. Ann Thorac Surg 2011, 92:998e1005
14.Bonvillain RW, Danchuk S, Sullivan DE, Betancourt AM, Semon JA, Eagle ME, Mayeux JP, Gregory AN, Wang G, Townley IK, Borg ZD, Weiss DJ, Bunnell BA: A nonhuman primate model of lung regeneration: detergent-mediated decellularization and initial in vitro recellularization with mesenchymal stem cells. Tissue Eng Part A 2012, 18:2437e2452
15.Atala A, Bauer SB, Soker S, Yoo JJ, Retik AB: Tissue-engineered autologous bladders for patients needing cystoplasty. Lancet 2006, 367:1241e1246
16.L’Heureux N, McAllister TN, de la Fuente LM: Tissue-engineered blood vessel for adult arterial revascularization. N Engl J Med 2007, 357:1451e1453
17.L’Heureux N, Paquet S, Labbe R, Germain L, Auger FA: A completely biological tissue-engineered human blood vessel. FASEB J 1998, 12:47e56
18.Gillies AR, Smith LR, Lieber RL, Varghese S: Method for decellularizing skeletal muscle without detergents or proteolytic enzymes. Tissue Eng Part C Methods 2011, 17:383e389
19.Mertsching H, Schanz J, Steger V, Schandar M, Schenk M, Hansmann J, Dally I, Friedel G, Walles T: Generation and transplantation of an autologous vascularized bioartificial human tissue. Transplantation 2009, 88:203e210
20.Mertsching H, Walles T, Hofmann M, Schanz J, Knapp WH: Engineering of a vascularized scaffold for artificial tissue and organ generation. Biomaterials 2005, 26:6610e6617
21.Macchiarini P, Jungebluth P, Go T, Asnaghi MA, Rees LE, Cogan TA, Dodson A, Martorell J, Bellini S, Parnigotto PP, Dickinson SC, Hollander AP, Mantero S, Conconi MT, Birchall MA: Clinical transplantation of a tissue-engineered airway. Lancet 2008, 372:2023e2030
22.Macchiarini P, Walles T, Biancosino C, Mertsching H: First human transplantation of a bioengineered airway tissue. J Thorac Cardiovasc Surg 2004, 128:638e641
23.Asnaghi MA, Jungebluth P, Raimondi MT, Dickinson SC, Rees LEN, Go T, Cogan TA, Dodson A, Parnigotto PP, Hollander AP, Birchall MA, Conconi MT, Macchiarini P, Mantero S: A double-chamber rotating bioreactor for the development of tissue-engineered hollow organs: from concept to clinical trial. Biomaterials 2009, 30:5260e5269
24.Sullivan DC, Mirmalek-Sani SH, Deegan DB, Baptista PM, Aboushwareb T, Atala A, Yoo JJ: Decellularization methods of porcine kidneys for whole organ engineering using a high-throughput
system. Biomaterials 2012, 33:7756e7764
25. Orlando G, Farney AC, Iskandar SS, Mirmalek-Sani SH, Sullivan DC, Moran E, AbouShwareb T, De Coppi P, Wood KJ, Stratta RJ, Atala A, Yoo JJ, Soker S: Production and implantation of renal extracellular matrix scaffolds from porcine kidneys as a platform for renal bioengineering investigations. Ann Surg 2012, 256: 363e370
26.Baptista PM, Siddiqui MM, Lozier G, Rodriguez SR, Atala A, Soker S: The use of whole organ decellularization for the generation of a vascularized liver organoid. Hepatology 2011, 53:604e617
27.Barakat O, Abbasi S, Rodriguez G, Rios J, Wood RP, Ozaki C, Holley LS, Gauthier PK: Use of decellularized porcine liver for engineering humanized liver organ. J Surg Res 2012, 173:e11ee25
28.Shupe T, Williams M, Brown A, Willenberg B, Petersen BE: Method for the decellularization of intact rat liver. Organogenesis 2010, 6: 134e136
29.Soto-Gutierrez A, Zhang L, Medberry C, Fukumitsu K, Faulk D, Jiang H, Reing J, Gramignoli R, Komori J, Ross M, Nagaya M, Lagasse E, Stolz D, Strom SC, Fox IJ, Badylak SF: A whole-organ regenerative medicine approach for liver replacement. Tissue Eng Part C Methods 2011, 17:677e686
30.Yagi H, Fukumitsu K, Fukuda K, Kitago M, Shinoda M, Obara H, Itano O, Kawachi S, Tanabe M, Coudriet GM, Piganelli JD, Gilbert TW, Soto-Gutierrez A, Kitagawa Y: Human-scale wholeorgan bioengineering for liver transplantation: a regenerative medicine approach. Cell Transplant 2013, 22:231e242
31.Keane TJ, Londono R, Turner NJ, Badylak SF: Consequences of ineffective decellularization of biologic scaffolds on the host response. Biomaterials 2012, 33:1771e1781
32.Brown BN, Valentin JE, Stewart-Akers AM, McCabe GP, Badylak SF: Macrophage phenotype and remodeling outcomes in response to biologic scaffolds with and without a cellular component. Biomaterials 2009, 30:1482e1491
33.Anderson JM, Charles W Jr, Antonios G, Larry V, Langer R: Biocompatibility of tissue engineered implants. Frontiers in Tissue Engineering. Oxford, Elsevier Science, 1998. pp 152-165
34.Brown BN, Londono R, Tottey S, Zhang L, Kukla KA, Wolf MT, Daly KA, Reing JE, Badylak SF: Macrophage phenotype as
564 |
ajp.amjpathol.org - The American Journal of Pathology |

Decellularized Porcine Liver
a predictor of constructive remodeling following the implantation of biologically derived surgical mesh materials. Acta Biomater 2012, 8: 978e987
35.Porcheray F, Viaud S, Rimaniol AC, Leone C, Samah B, DereuddreBosquet N, Dormont D, Gras G: Macrophage activation switching: an asset for the resolution of inflammation. Clin Exp Immunol 2005, 142:481e489
36.Stout RD, Jiang C, Matta B, Tietzel I, Watkins SK, Suttles J: Macrophages sequentially change their functional phenotype in response to changes in microenvironmental influences. J Immunol 2005, 175:342e349
37.Stout RD, Suttles J: Immunosenescence and macrophage functional plasticity: dysregulation of macrophage function by age-associated microenvironmental changes. Immunol Rev 2005, 205:60e71
38.Mikos AG, McIntire LV, Anderson JM, Babensee JE: Host response to tissue engineered devices. Adv Drug Deliv Rev 1998, 33:111e139
39.Vanderrest M, Garrone R: Collagen family of proteins. FASEB J 1991, 5:2814e2823
40.Bancroft JD, Gamble M: Theory and Practice of Histological Techniques. ed 5. Edinburgh, UK, Churchill Livingstone Press, 2002
41.Prophet EB Armed Forces Institute of Pathology: Laboratory Methods in Histotechnology. Washington, DC, American Registry of Pathology, 1992
42.Ji R, Zhang N, You N, Li Q, Liu W, Jiang N, Liu J, Zhang H, Wang D, Tao K, Dou K: The differentiation of MSCs into functional hepatocyte-like cells in a liver biomatrix scaffold and their transplantation into liver-fibrotic mice. Biomaterials 2012, 33:8995e9008
43.Wang Y, Cui CB, Yamauchi M, Miguez P, Roach M, Malavarca R, Costello MJ, Cardinale V, Wauthier E, Barbier C, Gerber DA, Alvaro D, Reid LM: Lineage restriction of human hepatic stem cells
to mature fates is made efficient by tissue-specific biomatrix scaffolds. Hepatology 2011, 53:293e305
44.Skardal A, Smith L, Bharadwaj S, Atala A, Soker S, Zhang YY: Tissue specific synthetic ECM hydrogels for 3-D in vitro maintenance of hepatocyte function. Biomaterials 2012, 33:4565e4575
45.Block GD, Locker J, Bowen WC, Petersen BE, Katyal S, Strom SC, Riley T, Howard TA, Michalopoulos GK: Population expansion, clonal growth, and specific differentiation patterns in primary cultures of hepatocytes induced by HGF/SF, EGF and TGF alpha in a chemically defined (HGM) medium. J Cell Biol 1996, 132:1133e1149
46.Sarathchandra P, Smolenski RT, Yuen AHY, Chester AH, Goldstein S, Heacox AE, Yacoub MH, Taylor PM: Impact of gamma-
irradiation on extracellular matrix of porcine pulmonary valves. J Surg Res 2012, 176:376e385
47.Kocgozlu L, Rabineau M, Koenig G, Haikel Y, Schaaf P, Freund JN, Voegel JC, Lavalle P, Vautier D: The control of chromosome segregation during mitosis in epithelial cells by substrate elasticity. Biomaterials 2012, 33:798e809
48.Engler AJ, Sen S, Sweeney HL, Discher DE: Matrix elasticity directs stem cell lineage specification. Cell 2006, 126:677e689
49.Rosario DJ, Reilly GC, Ali Salah E, Glover M, Bullock AJ, Macneil S: Decellularization and sterilization of porcine urinary bladder matrix for tissue engineering in the lower urinary tract. Regen Med 2008, 3: 145e156
50.Badylak SF, Freytes DO, Gilbert TW: Extracellular matrix as a biological scaffold material: structure and function. Acta Biomater 2009, 5:1e13
51.Anderson JM: Mechanisms of inflammation and infection with implanted devices. Cardiovasc Pathol 1993, 2:S33eS41
The American Journal of Pathology - ajp.amjpathol.org |
565 |