
Met_OrgSynthesis
.pdf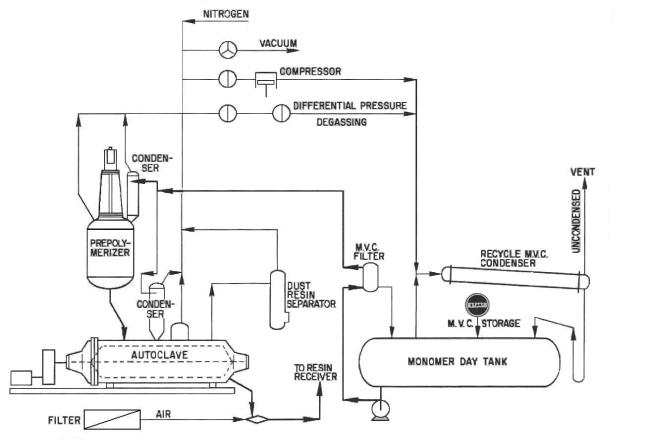
2.Look through the text to find the English equivalents for the following Russian words and word combinations.
Производство поливинилхлоридных смол, двухэтапный процесс, подряд, жидкая среда, образование шариков, оборудованный, размер частицы, форма шарика (бусинки), семя (зародыш), эффективное перемешивание, порошкообразный, в конце цикла, дальнейшая полимеризация, спецификационные характеристики, капитальные расходы, обезвоживание, непрерывная программа, автоматическое управление, приводить к.
3.Read the text and translate it into Russian.
4.Describe the process of PVC resins manufacture.
Fig. 6. Ethylene and butylene production scheme
69
PART III SUPPLEMENTARY READING
TEXT 1. METHANE
Methane is a chemical compound with the molecular formula CH4. It is the simplest alkane, and the principal component of natural gas. Methane's bond angles are 109.5 degrees. Burning methane in the presence of oxygen produces carbon dioxide and water.
The relative abundance of methane and its clean burning process makes it a very attractive fuel. However, because it is a gas at normal temperature and pressure, methane is difficult to transport from its source. In its natural gas form, it is generally transported in bulk by pipeline or LNG carriers; few countries still transport it by truck.
Methane was discovered and isolated by Alessandro Volta between 1776 and 1778 when studying marsh gas from Lake Maggiore.
Methane is a relatively potent greenhouse gas with a high global warming potential of 72 (averaged over 20 years) or 25 (averaged over 100 years). Methane in the atmosphere is eventually oxidized, producing carbon dioxide and water. As a result, methane in the atmosphere has a half life of seven years.
The abundance of methane in the Earth's atmosphere in 1998 was 1745 parts per billion, up from 700 ppb in 1750. In the same time period, CO2 increased from 278 to 365 parts per million. The radiative forcing effect due to this increase in methane abundance is about one-third of that of the CO2 increase. In addition, there is a large, but unknown, amount of methane in methane clathrates in the ocean floors. The Earth's crust contains huge amounts of methane. Large amounts of methane are produced anaerobically by methanogenesis. Other sources include mud volcanoes, which are connected with deep geological faults, and livestock (primarily cows) from enteric fermentation.
Methane is a significant contributor to global warming and the Kyoto protocol seeks to regulate its production.
Properties
Methane is the major component of natural gas, about 87% by volume. At room temperature and standard pressure, methane is a colorless, odorless gas; the smell characteristic of natural gas as used in homes is an artificial safety measure caused by the addition of an odorant, often methanethiol or ethanethiol. Methane has a boiling point of −161°C at a pressure of one atmosphere. As a gas it is flammable only over a narrow range of concentrations (5–15%) in air. Liquid methane does not burn unless subjected to high pressure (normally 4–5 atmospheres).
Methane is not toxic; however, it is highly flammable and may form explosive mixtures with air. Methane is violently reactive with oxidizers, halogens, and some halogen-containing compounds. Methane is also an asphyxiant and may displace oxygen in an enclosed space.
70
Reactions of methane
Main reactions with methane are: combustion, steam reforming to syngas, and halogenation. In general, methane reactions are hard to control. Partial oxidation to methanol, for example, is difficult to achieve; the reaction typically progresses all the way to carbon dioxide and water.
Combustion. In the combustion of methane, several steps are involved: Methane is believed to form a formaldehyde (HCHO or H2CO). The
formaldehyde gives a formyl radical (HCO), which then forms carbon monoxide (CO). The process is called oxidative pyrolysis:
CH4 + O2 → CO + H2 + H2O
Following oxidative pyrolysis, the H2 oxidizes, forming H2O, replenishing the active species, and releasing heat. This occurs very quickly, usually in significantly less than a millisecond.
2H2 + O2 →2H2O
Finally, the CO oxidizes, forming CO2 and releasing more heat. This process is generally slower than the other chemical steps, and typically requires a few to several milliseconds to occur.
2CO + O2 →2CO2
The result of the above is the following total equation:
CH4(g) + 2O2(g) → CO2(g) + 2H2O(l) + 890 kJ/mol
where bracketed "g" stands for gaseous form and bracketed "l" stands for liquid
form.
Hydrogen activation. The strength of the carbon-hydrogen covalent bond in methane is among the strongest in all hydrocarbons, and thus its use as a chemical feedstock is limited. Despite the high activation barrier for breaking the C–H bond, CH4 is still the principal starting material for manufacture of hydrogen in steam reforming. The search for catalysts which can facilitate C–H bond activation in methane and other low alkanes is an area of research with considerable industrial significance.
Reactions with halogens. Methane reacts with all halogens given appropriate conditions, as follows:
CH4 + X2 → CH3X + HX
where X is a halogen: fluorine (F), chlorine (Cl), bromine (Br), or iodine (I). This mechanism for this process is called free radical halogenation.
71
Uses
Methane is important for electrical generation by burning it as a fuel in a gas turbine or steam boiler. Compared to other hydrocarbon fuels, burning methane produces less carbon dioxide for each unit of heat released. At about 891 kJ/mol, methane's combustion heat is lower than any other hydrocarbon; but a ratio with the molecular mass (16.0 g/mol) divided by the heat of combustion (891 kJ/mol) shows that methane, being the simplest hydrocarbon, produces more heat per mass unit than other complex hydrocarbons. In many cities, methane is piped into homes for domestic heating and cooking purposes. In this context it is usually known as natural gas, and is considered to have an energy content of 39 megajoules per cubic meter, or 1,000 BTU per standard cubic foot.
Research is being conducted by NASA on methane's potential as a rocket fuel. One advantage of methane is that it is abundant in many parts of the solar system and it could potentially be harvested in situ, providing fuel for a return journey.
Current methane engines in development produce a thrust of 7,500 pounds, which is far from the seven million pounds needed to launch the space shuttle. Instead, such engines will most likely propel voyages from our moon or send robotic expeditions to other planets in the solar system.
Recently methane emitted from coal mines has been successfully converted to electricity.
Industrial uses
Methane is used in industrial chemical processes and may be transported as a refrigerated liquid (liquefied natural gas, or LNG). While leaks from a refrigerated liquid container are initially heavier than air due to the increased density of the cold gas, the gas at ambient temperature is lighter than air. Gas pipelines distribute large amounts of natural gas, of which methane is the principal component.
In the chemical industry, methane is the feedstock of choice for the production of hydrogen, methanol, acetic acid, and acetic anhydride. When used to produce any of these chemicals, methane is first converted to synthesis gas, a mixture of carbon monoxide and hydrogen, by steam reforming. In this process, methane and steam react on a nickel catalyst at high temperatures (700–1100 °C).
The ratio of carbon monoxide to hydrogen in synthesis gas can then be adjusted via the water gas shift reaction to the appropriate value for the intended purpose.
CO + H2O → CO2 + H2
Less significant methane-derived chemicals include acetylene, prepared by passing methane through an electric arc, and the chloromethanes (chloromethane, dichloromethane, chloroform, and carbon tetrachloride), produced by reacting methane with chlorine gas. However, the use of these chemicals is declining. Acetylene is replaced by less costly substitutes, and the use of chloromethanes is diminishing due to health and environmental concerns.
72
TEXT 2. ETHYLENE
Ethylene (IUPAC name: ethene) is the chemical compound with the formula C2H4. It is the simplest alkene. Because it contains a carbon-carbon double bond, ethylene is called an unsaturated hydrocarbon or an olefin. It is extremely important in industry and also has a role in biology as a hormone. Ethylene is the most produced organic compound in the world; global production of ethylene exceeded 107 million metric tonnes in 2005.
From 1795 on, ethylene was referred as the olefiant gas (oil-making gas), because it combined with chlorine to produce the oil of the Dutch (1,2- dichloroethane). Ethylene was first synthesized in 1795 by a collaboration of four Dutch chemists.
In 1866, the German chemist August Wilhelm von Hofmann proposed a system of hydrocarbon nomenclature in which the suffixes -ane, -ene, -ine, -one, and -une were used to denote the hydrocarbons with 0, 2, 4, 6, and 8 fewer hydrogens than their parent alkane. In this system, ethylene became ethene. Hofmann's system eventually became the basis for the Geneva nomenclature approved by the International Congress of Chemists in 1892, which remains at the core of the IUPAC nomenclature. However, by that time, the name ethylene was deeply entrenched, and it remains in wide use today, especially in the chemical industry.
The 1979 IUPAC nomenclature rules made an exception for retaining the nonsystematic name ethylene, however, this decision was reversed in the 1993 rules so the correct name is now ethene.
Uses
Approximately 80% of ethylene used in the United States and Europe is used to create ethylene oxide, ethylene dichloride, and polyethylene. In smaller quantities, ethylene is used as an anesthetic agent (in an 85% ethylene/15% oxygen ratio), to hasten fruit ripening, as well as a welding gas.
Polyethylenes of various density and melt flow account for more than 50% of world ethylene demand. The primary use of polyethylene is in film applications for packaging, carrier bags and trash liners. Other applications include injection moulding, pipe extrusion, wire and cable sheathing and insulation, as well as extrusion coating of paper and cardboard.
Ethylene derivatives include: ethylene oxide, styrene monomer (via ethyl benzene) and linear higher olefins.
Ethylene oxide is a key raw material in the production of surfactants and detergents. It is also used to manufacture ethylene glycols, which are in turn used in packaging and textiles, and to make glycol ether solvents.
Styrene monomer is used principally in polystyrene for packaging and insulation, as well as in styrene butadiene rubber for tires and footwear.
Linear higher olefins are used as base materials for the manufacture of detergents, plasticisers, synthetic lubricants and additives, but also as co-monomers in the production of polyethylenes.
73
Production
Ethylene is produced in the petrochemical industry by steam cracking. In this process, gaseous or light liquid hydrocarbons are heated to 750–950 °C, inducing numerous free radical reactions followed by immediate quench to freeze the reactions. This process converts large hydrocarbons into smaller ones and introduces unsaturation. Ethylene is separated from the resulting complex mixture by repeated compression and distillation. In a related process used in oil refineries, high molecular weight hydrocarbons are cracked over zeolite catalysts. Heavier feedstocks, such as naphtha and gas oils require at least two "quench towers" downstream of the cracking furnaces to recirculate pyrolysis-derived gasoline and process water. The areas of an ethylene plant are:
1.steam cracking furnaces;
2.primary and secondary heat recovery with quench;
3.a dilution steam recycle system between the furnaces and the quench system;
4.primary compression of the cracked gas (3 stages of compression);
5.hydrogen sulfide and carbon dioxide removal (acid gas removal);
6.secondary compression (1 or 2 stages);
7.drying of the cracked gas;
8.cryogenic treatment;
9.all of the cold cracked gas stream goes to the demethanizer tower. The overhead stream from the demethanizer tower consists of all the hydrogen and methane that was in the cracked gas stream. Different methods of cryogenically treating this overhead stream results in the separation of the hydrogen and the methane.
This usually involves liquid methane at a temperature around −250°F (−156.7 °C). Complete recovery of all the methane is critical to the economical operation of an ethylene plant. Often one or two Turboexpanders are used for Methane recovery from the demethanizer overhead stream.
10.the bottom stream from the demethanizer tower goes to the deethanizer tower. The overhead stream from the deethanizer tower consists of all the C2,'s that were in the cracked gas stream. The C2's then go to a C2 splitter. The product ethylene is taken from the overhead of the tower and the ethane coming from the bottom of the splitter is recycled to the furnaces to be cracked again;
11.the bottom stream from the deethanizer tower goes to the depropanizer tower. The overhead stream from the depropanizer tower consists of all the C3's that were in the cracked gas stream. Prior to sending the C3's to the C3 splitter this stream is hydrogenated in order to react out the methylacetylene and propadiene. Then this stream is sent to the C3 splitter. The overhead stream from the C3 splitter is product propylene and the bottom stream from the C3 splitter is propane which can be sent back to the furnaces for cracking or used as fuel.
12.The bottom stream from the depropanizer tower is fed to the debutanizer tower. The overhead stream from the debutanizer is all of the C4's that was in the cracked gas stream. The bottom stream from the debutanizer consists of everything in the cracked gas stream that is C5 or heavier. This could be called a light pyrolysis gasoline.
74
Since the production of ethylene is energy intensive, much effort has been dedicated recovering heat from the gas leaving the furnaces. Most of the energy recovered from the cracked gas is used to make high pressure (1200 psig) steam. This steam is in turn used to drive the turbines for compressing cracked gas, the propylene refrigeration compressor, and the ethylene refrigeration compressor. An ethylene plant, once running, does not need to import any steam to drive its steam turbines. A typical world scale ethylene plant (about 1.5 billion pounds of ethylene per year) uses a 45,000 horsepower (34,000 kW) cracked gas compressor, a 30,000 horsepower (22,000 kW) propylene compressor, and a 15,000 horsepower (11,000 kW) ethylene compressor.
When starting an ethylene plant it is important to start the cooling systems in the proper order. The cooling systems consist of Cooling Tower Water (CTW); propylene refrigeration with four or five different levels or stages. Each level corresponds to a particular pressure and temperature; and three or four stages of ethylene regfrigeration. The CTW must be started first because the propylene system needs it to condense propylene and the ethylene refrigeration systems needs it to desuperheat high pressure ethylene. The propylene system must start next because the ethylene system needs high pressure propylene for desuperheating the high pressure ethylene stage and the low pressure propylene stage for condensing the high pressure ethylene. While the ethylene plant is running, the plant can continue to run for a time if the ethylene refrigeration compressor shuts down. However, if the propylene compressor shuts down the whole plant must be shut down immediately.
Manufacturers
The world's largest ethylene complex is located in Iran. The complex will produce over 1,320 million metric tons of ethylene a year, it could also produce 306,000 tons of propylene, 600,000 tons of a variety of linear light and heavy polyethylene, 300,000 tons of polypropylene, 443,000 tons of ethylene glycol, and 245,000 tons of different 4C compounds annually.
Laboratory preparation
Ethylene can be conveniently produced in the laboratory by distilling absolute ethanol with an excess of concentrated sulfuric acid and washing the distillate vapor stream in an aqueous solution of sodium hydroxide to remove the sulfur dioxide contaminant.
Chemical reactions
Ethylene is an extremely important building block in the petrochemical industry. A list of some major types of reactions includes: 1) Polymerization, 2) Oxidation, 3) Halogenation and Hydrohalogenation, 4) Alkylation, 5) Hydration, 6) Oligomerization, 7) Oxo-reaction.
Additions to double bond. Like most alkenes, ethylene reacts with halogens to produce halogenated hydrocarbons1,2-C2H4X2. It can also react with water to produce ethanol, but the rate at which this happens is very slow unless a suitable catalyst, such as phosphoric or sulfuric acid, is used. Under high pressure, and, in the presence of a catalytic metal (platinum, rhodium, nickel), hydrogen will react with ethylene to form ethane.
75
Ethylene is used primarily as an intermediate in the manufacture of other chemicals in the synthesis of monomers. Ethylene can be chlorinated to produce 1,2- dichloroethane (ethylene dichloride). This can be converted to vinyl chloride, the monomer precursor to plastic polyvinyl chloride, or combined with benzene to produce ethylbenzene, which is used in the manufacture of polystyrene, another important plastic. Ethylene is more reactive than alkanes because of two reasons:
1.It has a double bond, one called the π-bond(pi) and one called the σ-bond (sigma), where the π-bond is weak and the σ-bond is strong. The presence of the π- bond makes it a high energy molecule. Thus bromine water decolourises readily when it is added to ethylene.
2.High electron density at the double bond makes it react readily. It is broken in an addition reaction to produce many useful products.
Polymerization. Ethylene polymerizes to produce polyethylene, also called polyethene or polythene, the world's most widely-used plastic.
Major polyethylene product groups are low density polyethylene, high density polyethylene, polyethylene copolymers, as well as ethylene-propylene co- & terpolymers.
Oxidation. Ethylene is oxidized to produce ethylene oxide, which is hydrolysed to ethylene glycol. It is also a precursor to vinyl acetate.
Ethylene undergoes oxidation by palladium to give acetaldehyde. This conversion was at one time a major industrial process. The process proceeds via the initial complexation of ethylene to a Pd(II) center.
Major intermediates of the oxidation of Ethylene are ethylene oxide, acetaldehyde, vinyl acetate and ethylene glycol. Some of the products made from these intermediates are: polyesters, polyurethane, morpholine, ethanolamines, aspirin and glycol ethers.
Halogenation and hydrohalogenation. Major intermediates from the halogenation and hydrohalogenation of ethylene include: ethylene dichloride, ethyl chloride and ethylene dibromide. Some products in this group are: polyvinyl chloride, trichloroethylene, perchloroethylene, methyl chloroform, polyvinylidiene chloride and copolymers, and ethyl bromide.
Alkylation. Major chemical intermediates from the alkylation of ethylene include: ethylbenzene, ethyl toluene, ethyl anilines, 1,4-hexadiene and aluminium alkyls. Products of these intermediates include polystyrene, unsaturated polyesters and ethylene-propylene terpolymers.
Hydration. Ethanol is the primary intermediate of the hydration of ethylene. Important products from ethanol are: ethylamines, acetaldehyde, and ethyl acetate.
Oligomerization. The primary products of the Oligomerization of ethylene are alpha-olefins and linear primary alcohols. These are used as plasticizers and surfactants.
Oxo-reaction. The Oxo-reaction of ethylene results in propionaldehyde with its primary products of propionic acid and n-propyl alcohol.
Ethylene is useful in organic synthesis. Representative reactions include DielsAlder additions, ene reaction, and arene alkylation.
76
TEXT 3. ACETYLENE
Acetylene (IUPAC name: ethyne) is the chemical compound with the formula HC2H. It is a hydrocarbon and the simplest alkyne. This colourless gas is widely used as a fuel and a chemical building block. It is unstable in pure form and thus is usually handled as a solution.
As an alkyne, acetylene is unsaturated because its two carbon atoms are bonded together in a triple bond. The carbon-carbon triple bond places all four atoms in the same straight line, with CCH bond angles of 180°.
Acetylene was discovered in 1836 by Edmund Davy who identified it as a "new carburet of hydrogen." It was rediscovered in 1860 by French chemist Marcellin Berthelot, who coined the name "acetylene." Berthelot was able to prepare this gas by passing vapours of organic compounds (methanol, ethanol, etc) through a red-hot tube and collecting the effluent. He also found acetylene was formed by sparking electricity through mixed cyanogen and hydrogen gases. He was also able to form acetylene directly by combining pure hydrogen with carbon using electrical discharge of a carbon arc.
Preparation
Nowadays acetylene is mainly manufactured by the partial combustion of methane or by the cracking of hydrocarbons. Approximately 400M kg are produced this way annually. Acetylene is also an undesirable byproduct of cracking reactions in refineries, in the process of the production of ethylene. This undesired side reaction represents the largest scale production of acetylene. Acetylene is problematic because of its explosive character and its ability to poison Ziegler-Natta catalysts. It is selectively hydrogenated into ethylene, usually using Pd-Ag catalysts.
Alternatively acetylene can be prepared by mixing calcium carbide and water, a reaction was discovered by Friedrich Wohler in 1862:
CaC2 + 2H2O → Ca(OH)2 + C2H2
Calcium carbide production requires extremely high temperatures, ~2000 degrees Celsius, necessitating the use of an electric arc furnace. In the US, this reaction was an important part of the late-1800s revolution in chemistry enabled by the massive hydroelectric power project at Niagara Falls.
Reactions
Approximately 80 percent of the acetylene produced annually in the United States is used in chemical synthesis. One new application is the conversion of acetylene to ethylene for use in making a variety of polyethylene plastics. An important reaction of acetylene is its combustion, the basis of the acetylene welding technologies. Otherwise, its major applications involve its converstion to acrylic acid derivatives. Acetylides with many metal ions by reactions of with solutions of their salts. Several, e.g., silver acetylide and copper acetylide, are powerful explosives.
77
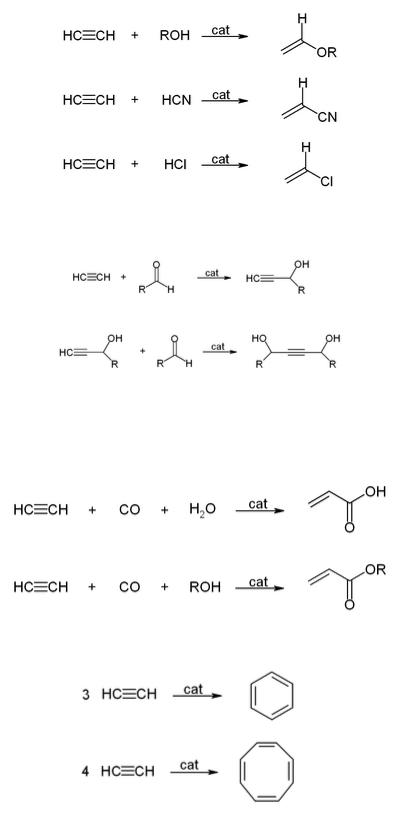
Copper acetylide is also formed by reacting acetylene with metallic copper or its alloys; these materials are therefore unsuitable for installations for handling acetylene.
Walter Reppe discovered that in the presence of metal catalysts, acetylene can react to give a wide range of industrially significant chemicals.
With alcohols, hydrogen cyanide, hydrogen chloride, or carboxylic acids to give vinyl compounds:
With aldehydes to give ethynyl diols.
1,4-Butynediol is produced industrially in this way from formaldehyde and acetylene.
With carbon monoxide to give acrylic acid, or acrylic esters, which can be used to produce acrylic glass.
Cyclicization to give benzene and cyclooctatetraene:
78