
- •Table of Contents
- •Front matter
- •Copyright
- •Preface to the twelfth edition
- •Preface to the eleventh edition
- •Preface to the tenth edition
- •Acknowledgements
- •Chapter 1. Introduction to regional anatomy
- •2. Upper limb
- •Part two. Shoulder
- •Part three. Axilla
- •Part four. Breast
- •Part five. Anterior compartment of the arm
- •Part six. Posterior compartment of the arm
- •Part eight. Posterior compartment of the forearm
- •Part nine. Wrist and hand
- •Part ten. Summary of upper limb innervation
- •Part eleven. Summary of upper limb nerve injuries
- •Part twelve. Osteology of the upper limb
- •Chapter 3. Lower limb
- •Part two. Medial compartment of the thigh
- •Part three. Gluteal region and hip joint
- •Part four. Posterior compartment of the thigh
- •Part five. Popliteal fossa and knee joint
- •Part six. Anterior compartment of the leg
- •Part seven. Dorsum of the foot
- •Part eight. Lateral compartment of the leg
- •Part nine. Posterior compartment of the leg
- •Part ten. Sole of the foot
- •Part eleven. Ankle and foot joints
- •Part twelve. Summary of lower limb innervation
- •Part thirteen. Summary of lower limb nerve injuries
- •Part fourteen. Osteology of the lower limb
- •Chapter 4. Thorax
- •Part one. Body wall
- •Part three. Thoracic cavity
- •Part five. Anterior mediastinum
- •Part eight. Pleura
- •Chapter 5. Abdomen
- •Part two. Abdominal cavity
- •Part nine. Spleen
- •Part eleven. Kidneys, ureters and suprarenal glands
- •Part twenty. Female urogenital region
- •Chapter 6. Head and neck and spine
- •Part three. Prevertebral region
- •Part eleven. Mouth and hard palate
- •Part fifteen. Lymph drainage of head and neck
- •Part twenty-two. Vertebral canal
- •Chapter 7. Central nervous system
- •Part two. Brainstem
- •Part three. Cerebellum
- •Part four. Spinal cord
- •Part five. Development of the spinal cord and brainstem nuclei
- •Chapter 8. Osteology of the skull and hyoid bone
- •Part two. Hyoid bone
- •Biographical notes
- •Index
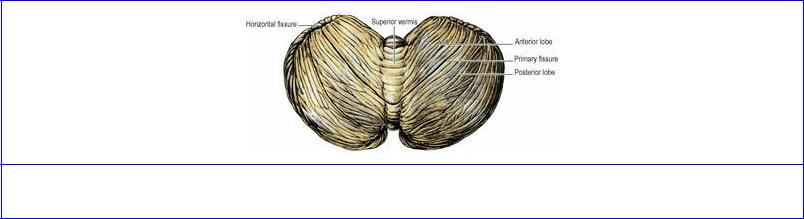
Part three. Cerebellum
The cerebellum accounts for about 10% of the total weight of the brain and occupies the posterior cranial fossa. It consists of two hemispheres united in the midline by the vermis (Fig. 7.28). Three peduncles connect each hemisphere to the three parts of the brainstem (Fig. 7.20). The superior peduncle enters the midbrain, the middle peduncle consists of the transverse fibres of the pons and the inferior peduncle connects with the medulla. The ventral surface of the vermis lies on the superior medullary velum and the roof of the medullary part of the fourth ventricle.
Figure 7.28 Cerebellum: superior aspect.
The cerebellum is bounded posteriorly by a convex border that lies below the attached margin of the tentorium cerebelli. From this border the superior surface slopes concavely upwards, in conformity with the shape of the tentorium. The posteroinferior surface is boldly convex below the posterior border and occupies the concavity of the occipital bone.
The surface of the cerebellum is indented by fine slitlike sulci, between which lie more or less parallel folds or folia. In the main the folia and sulci lie transversely from side to side across the whole extent of the cerebellum.
A well-marked groove, the horizontal fissure (of no functional significance), extends around the posterolateral border of each hemisphere and divides the cerebellum into superior and inferior halves. On the superior surface, is a much shallower groove, the primary fissure. The hemispheres consist of a small anterior lobe on the superior surface in front of the primary fissure and a large posterior lobe comprising the rest of the hemisphere behind the primary fissure (Fig. 7.28). The tonsil is a roughly spherical lobule on the inferior aspect of the posterior lobe. The tonsil may be displaced down through the foramen magnum in conditions of severe raised intracranial pressure or in congenital craniovertebral and hindbrain malformations.
The vermis consists of superior and inferior parts. The superior vermis forms a ridge between the hemispheres (anterior lobe) on the superior surface. Its anterior part, the lingula, lies in contact with the superior medullary velum (Fig. 7.29). On the inferior surface of the posterior lobe there is a deep groove, the vallecula, between the hemispheres. The inferior vermis lies in the groove and consists of the tuber vermis, pyramid, uvula and nodule. The nodule lies on the roof of the fourth ventricle and projecting laterally from each side of the nodule is a slender band of white matter whose bulbous extremity, capped with grey matter, can be seen from in front, lying in the angle between cerebellum and pons. This is the flocculus; the choroid plexus of the fourth ventricle projects just beside it (Fig. 7.18). The two flocculi and the nodule form the flocculonodular lobe.
In functional terms the cerebellum is divided into a corpus cerebelli (which has afferents from the spinal cord and trigeminal nuclei, and inputs from the pontine nuclei) and a flocculonodular lobe (which, with the lingula, has connections with the vestibular nuclei). Lesions of the latter part lead to disturbances of equilibrium with no alteration of spinal reflexes. The anterior lobe and the pyramid are the main recipients of spinal and trigeminal afferents. Lesions of this part cause disturbances of postural mechanisms with increased muscle reflexes. Cerebropontine connections are relayed by the pontine nuclei via the middle peduncle to the posterior lobe, tuber vermis and uvula. Lesions of these regions result in hypotonia, diminished or pendulum muscle jerks, intention tremor, clumsy movements and nystagmus (oscillatory movement of the eye).
The essential function of the cerebellum is the coordination of movement. Cerebellar lesions do not cause paralysis, but disturbances of movement and balance.
Like the cerebrum, the cerebellum is surfaced with a cortex of grey matter, with the white matter internal. Unlike the cerebral cortex, the cerebellar cortex consists of three layers. Embedded within the white matter are four pairs of nuclei. The most lateral, the dentate nucleus, is the largest. It forms a crenated crescent, resembling the inferior olivary nucleus in the medulla, open towards the superior peduncle. Its main connections are cerebropontocerebellar, and its efferent fibres leave the hilum and pass to the contralateral red nucleus, thalamus and cerebral cortex.
Cerebellar peduncles and connections
The superior and middle peduncles are simple; the inferior peduncle contains a great mixture of fibres.
The superior peduncle contains efferent fibres, passing from the dentate nucleus to the red nucleus, thalamus and cortex of the opposite side. It also contains the afferent anterior spinocerebellar tract, and tectocerebellar fibres from the midbrain.
The middle peduncle contains afferent fibres from the pontine nuclei of the opposite side.
T h e inferior peduncle contains the efferent cerebellovestibular tract and the afferent vestibulocerebellar tract, both connected to the vestibular nuclei of the same side. It also contains the afferent posterior spinocerebellar and cuneocerebellar tracts (the latter consisting of proprioception fibres from the upper limb), and the olivocerebellar tract from the olivary nuclei of the opposite side.
Blood supply
Two arteries supply the large convex posteroinferior surface and one artery supplies the small upper surface of each cerebellar hemisphere. They anastomose with each other on the cerebellar surface, but their perforating branches into the cerebellum are, as elsewhere in the nervous system, end arteries.
The posterior inferior cerebellar artery is one of the most tortuous arteries in the body, and is the largest branch of the vertebral artery. It arises ventrally from the vertebral artery, near the lower end of the olive, and spirals back around the medulla below the hypoglossal rootlets and then between the rootlets of the glossopharyngeal and vagus nerves. It supplies the choroid plexus of the fourth ventricle and is distributed to the inferior vermis and the back of the cerebellar hemispheres. It
supplies, in passing, the adjacent part of the medulla as described on page 481.
The anterior inferior cerebellar artery arises from the basilar artery at the lower part of the pons and passes back on the inferior surface of the cerebellar hemisphere, supplying this surface and the adjacent flocculus. It may give rise to the labyrinthine artery if it has not arisen from the basilar.
The superior cerebellar artery arises near the termination of the basilar and passes laterally to wind around the cerebral peduncle below the fourth nerve. It is distributed over the superior surface of the cerebellum.
Venous drainage is from the surface of the cerebellum into the nearest available venous sinus of the dura mater. Thus the superior and posterior surfaces drain into the straight and transverse sinuses, inferior surfaces into the inferior petrosal, sigmoid and occipital sinuses.
Part four. Spinal cord
The spinal cord, or spinal medulla, is a cylinder, somewhat flattened from front to back, whose lower end tapers into a cone. Ventrally it possesses a deep midline groove, the anterior median fissure, and dorsally it shows a shallow posterior median sulcus, from which a posterior median septum of neuroglia extends into its substance.
In the fetus the spinal cord extends to the lower limit of the spinal dura mater at the level of S2 vertebra. The spinal dura continues to reach this level throughout life, but the spinal cord becomes relatively shorter, which is to say that the bony spinal column and the dura mater grow more rapidly than the spinal cord. Thus at birth the conus medullaris lies opposite L3 vertebra and does not reach its permanent level opposite L1 or L2 until about the age of 20 years. The spinal nerve roots, especially those of the lumbar and sacral segments, thus come to slope more and more steeply downwards.
The spinal cord possesses two symmetrical enlargements which occupy the segments of the limb plexuses: as the cervical enlargement (C5 to T1) for the brachial plexus and the lumbosacral enlargement (L2 to S3) for the lumbar and sacral plexuses. Their levels measured by vertebrae are, of course, quite different; the cervical enlargement is approximately opposite vertebrae C3 to T1, but the lumbosacral extends only from T9 to L1. Both enlargements are due to the greatly increased mass of motor cells in the anterior horns of grey matter in these situations.
The spinal meninges have been described on page 453.
Spinal nerve roots
The anterior and posterior roots of the spinal nerves unite within the intervertebral foramina. Within the subarachnoid space the nerve roots are attached to the spinal cord each by a series of rootlets. Each anterior root is formed by three or four rootlets which emerge irregularly along the anterolateral surface of the spinal cord. Each posterior root is formed by several rootlets, attached vertically to the posterolateral surface of the cord. A short distance from the cord the rootlets are combined into a single root (see Fig. 6.111, p. 454 ). The anterior and posterior roots pass from the cord to their appropriate intervertebral foramina, where each evaginates the dura mater separately before uniting to form the mixed spinal nerve. The ganglion on the posterior nerve root lies in the intervertebral foramen, within the tubular evagination of dura and arachnoid immediately proximal to the point of union of anterior and posterior nerve roots. However, the posterior root ganglia of cervical nerves lie partly lateral to the intervertebral foramina, behind and in contact with the vertebral artery (see Fig. 6.109, p. 452); the ganglion of the first cervical nerve, however, lies on the posterior arch of the atlas. For all levels from C1 to L1 vertebrae the anterior and posterior nerve roots pass in front of and behind the denticulate ligament respectively, and evaginate the dura mater between the denticulations (see Fig. 6.111, p. 454). In conformity with the shortness of the spinal cord, the lower a nerve root the more steeply it slopes down to the intervertebral foramen. The upper cervical roots are horizontal, the upper thoracic roots first slope down to their point of evagination of the meninges only to become kinked upwards at an angle to reach their foramen (Fig. 7.30). Below L1 vertebra the roots pass almost vertically downwards through the subarachnoid space, forming the cauda equina. The filum terminale (pia mater, see p. 453) extends down from the tip of the conus medullaris among the nerve roots of the cauda.

Figure 7.30 Upper thoracic spinal nerve roots, from behind, showing the upward angulation of the roots as they emerge from the spinal theca.
The roots of the spinal part of the accessory nerve emerge from the lateral surface of the upper five or six segments of the cord, behind the denticulate ligament. They unite into a single trunk which passes upwards through the foramen magnum into the cranium to join the cranial root (see Fig. 6.109 p. 452).
Internal structure
The spinal cord consists of a central mass of grey matter (cell bodies), in the form of a vertically grooved column surrounding the central canal, enclosed in a cylindrical mass of white matter (fibres) (Fig. 7.31). It is almost divided into two halves by the anterior median fissure and the posterior median septum. The septum extends forwards as far as the grey commissure (the central limb of the H in cross-section) which connects the grey matter of the right and left halves of the cord, and contains the central canal. This is the tiny downward continuation of the cavity of the fourth ventricle and like it lined by ependyma. It extends into the upper few millimetres of the filum terminale. The anterior fissure does not completely separate the white matter—a narrow white commissure lies anterior to the grey. On account of the shape of the grey matter and the attachment of nerve roots, the grey and white matter of the right and left halves is divisible into anterior, lateral and posterior parts, referred to as horns for the grey matter and columns for the white matter.
Figure 7.31 Cross-section of the spinal cord showing the main tracts and laminae of grey matter. The small medial vestibulospinal tract (not shown) lies close to the anterior median fissure of the
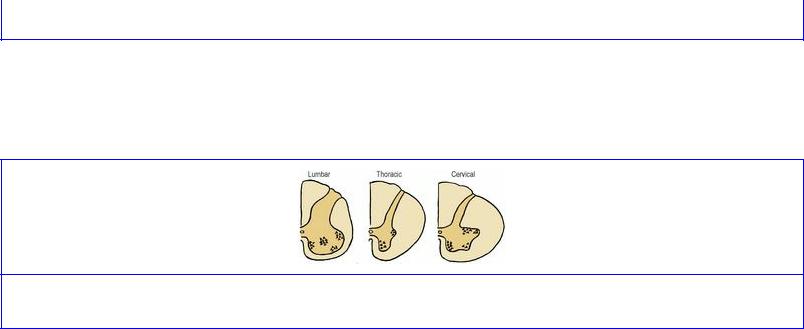
cervical cord.
The shapes of the grey horns and white columns in sections enables the three regions to be distinguished (Fig. 7.32). The anterior grey horns are largest in the cervical and lumbar regions and the posterior white columns largest in the cervical regions.
Figure 7.32 Cross-section of the spinal cord in the lumbar, thoracic and cervical regions.
Grey matter
The posterior horn stretches to the surface of the cord, but the anterior horn falls short of it. The cervical and lumbosacral enlargements are due to a great increase in the number of anterior horn cells, to provide fibres for the great nerve plexuses. The more medial anterior horn cells are concerned with the innervation of trunk musculature, with the more lateral cells supplying the limbs. The more ventral cells of the lateral group supply proximal limb muscles, and the more dorsal cells innervate the more distal limb muscles.
Between the limb enlargements, from segments T1 to L2, there is a small lateral horn, containing preganglionic sympathetic cell bodies (see Fig. 1.13C, p. 19). Their axons pass out in the anterior nerve roots and enter the spinal nerves from T1 to L2 which they leave in the white rami communicantes passing to the sympathetic trunk (see p. 19). A similar group of cells forms the small lateral horn in sacral segments 2–4; these are preganglionic parasympathetic cell bodies whose axons leave in those sacral anterior nerve roots and emerge distally from the sacral nerves as pelvic splanchnic nerves (see p. 282).
The cells of the grey matter in each half of the cord lie in specific functional groups or laminae, designated by the Roman numerals I to X (Fig. 7.31). Among the more important cell groups in the various laminae are those of lamina II which constitute the gelatinous substance; the cells of lamina V are a main source of anterolateral tract (spinothalamic and spinoreticular) fibres; lamina VII contains in its medial part the thoracic nucleus and laterally the thoracolumbar (sympathetic) and sacral (parasympathetic) lateral horn cells; other cells of lamina VII, together with those of lamina VIII, are interneurons involved in coordinating motor activity and projecting to lamina IX; lamina IX contains the α and γ motor neurons which innervate skeletal muscle.
White matter
In each half of the cord the posterior white column lies between the posterior median septum and the posterior grey horn. It is wholly occupied by the ascending fibres of the gracile and cuneate tracts, the pathways for touch and some associated sensations (see below).
The rest of the white matter forms the lateral and anterior white columns, the emerging anterior nerve roots providing a convenient dividing line between the two. Both these columns contain long ascending and descending tracts. In addition, short intersegmental fibres run up and down adjacent to the central grey matter, forming communications between segments. Except in the posterior columns, there is much intermingling of fibres and there are no sharp boundaries to tracts.
Afferent pathways
There are three possible destinations for all incoming fibres: the cortex of the opposite cerebral hemisphere, via thalamic relay, for conscious sensation; the cerebellum, for muscular coordination; and the brainstem or spinal cord, for reflex actions.
As a guiding principle it is usually stated that afferent impulses are conveyed to the cerebral cortex by three groups of neurons. While this is a convenient concept and accurate enough for the main longfibre components, it takes no account of the vast numbers of short interneurons in the neuronal pathways. The cell bodies of the first group of neurons (first neurons or first order neurons ) lie outside the central nervous system: in the posterior root ganglia of spinal nerves or the equivalent ganglia of cranial nerves. The cell bodies of the second neurons are in the spinal cord or brainstem, and those of the third neurons are in the thalamus.
Efferent pathways
For the control of the skeletal muscles supplied by spinal nerves, two main systems of neurons are involved. One can be called the direct corticospinal pathway, consisting essentially of two groups of neurons. The cell bodies of the first are in the cerebral cortex, and their fibres extend through the internal capsule and brainstem to the anterior horn cells of the cord; these are the corticospinal fibres. The corresponding corticonuclear fibres go to the motor nuclei of cranial nerves. These fibres (often called pyramidal; see p. 481) with their cortical cell bodies are known as the upper motor neurons. The second neurons are the anterior horn cells and their axons which end as the motor endplates on skeletal muscle fibres. These neurons (and those of the motor nuclei of cranial nerves) are called the lower motor neurons. This sequence does not take account of interneurons that participate in this pathway.
The other system involves a whole series of neurons on the way to anterior horn cells. For example, cortical cells may send their axons to pontine nuclei, from which fibres run to the cerebellum which in turn may project to the red, reticular, vestibular and olivary nuclei, all of which can communicate with the same anterior horn cells as pyramidal fibres. Other cortical fibres run to the basal nuclei (corpus striatum) and thalamus, with projections from them to the red, reticular and subthalamic nuclei and the substantia nigra. These brainstem nuclei can also be activated by reflex sensory pathways (e.g. light and sound). From some of these brainstem groups fibres descend to synapse with the same anterior horn cells that have received corticospinal fibres. None of these fibres has passed through the pyramid of the medulla (hence the name extrapyramidal) and because none has passed uninterruptedly from the cortex to anterior horn cells they are also called indirect corticospinal pathways.
Ascending tracts
The most important ascending tracts fall into two groups. Those in the lateral and anterior white
columns are concerned with pain (nociception) and temperature sensations, crude touch, and the sensations of itch, tickle and sexual orgasm. Here also are tracts to the cerebellum, for muscular coordination.
The posterior white column is wholly occupied by ascending fibres of the gracile and cuneate tracts. They are concerned with light (discriminative) touch, vibration sense, proprioception (muscle joint or kinaesthetic sense, the conscious appreciation of body position and movement), and the sense of fullness of the bladder and rectum. Their cell bodies lie in the posterior root ganglia of spinal nerves, and the fibres enter the cord from the medial side of the posterior nerve roots. Some branches from the incoming posterior root fibres are short and take part in segmental reflex arcs, but it is the long ascending fibres that form the tracts. The fibres from the lowest parts of the body lie nearest the midline, and incoming fibres are added progressively laterally, i.e. the column is laminated. In this way fibres from the perineum, lower limb and lower trunk form the gracile tract, and those from the upper trunk and upper limb form the more lateral cuneate tract. The two tracts end in the lower part of the medulla by synapsing with the cells of the gracile and cuneate nuclei respectively. The axons from these (second order) nuclei immediately decussate to form the medial lemniscus which runs through the brainstem to the thalamus. After relay there, the axons of the third group of neurons pass via the internal capsule to area SmI of the cerebral cortex.
Tests for the types of sensation transmitted by the posterior columns include those for light touch, such as gentle stroking of the skin with the fingers or cotton wool. Position sense is tested by the examiner passively moving finger or great toe joints with the patient's eyes closed, to see whether the direction of movement can be appreciated. If upper limb position sense is disturbed the patient cannot hold the outstretched limb and fingers steady with the eyes closed, nor perform the finger-nose test accurately. Similarly, lower limb position sense is tested by standing upright with the feet together and then closing the eyes. Without the visual sense to relate to the environment, there will be loss of balance with the eyes shut (Romberg's sign) if there is loss of this posterior column sense. For the same reason there will be difficulty with walking in the dark, or keeping the balance when washing the face with the eyes shut. These are examples of sensory ataxia. (With labyrinthine lesions or cerebellar ataxia there is little difference whether the eyes are open or shut.) Vibration sense, which is also conducted by the posterior columns, is tested by applying a vibrating tuning fork (128 Hz, middle C) to a bony prominence such as the lateral malleolus.
The two-point discrimination test is important because it not only involves the peripheral and central pathways, but the cerebral cortex as well. Two points 3 mm apart are normally distinguishable on the finger pads. Stereognostic sense (ability to appreciate the size and shape of objects in the hand) also requires the intact cortex.
The main ascending tract of the lateral and anterior white columns is the anterolateral tract. It is concerned with pain (nociception) and temperature sensations, crude touch, and the sensations of itch, tickle and sexual orgasm. It is a large bundle lying in front of the level of attachment of the denticulate ligament and extending into the anterior white column.
The pain fibres in peripheral nerves are of two kinds: small C fibres, unmyelinated and slowconducting, for the dull, aching type of pain (‘slow pain’), and Aδ fibres, thinly myelinated and fastconducting, for the sharp, pricking type of pain (‘fast pain’) and also for temperature conduction (see
p. 10). On entering the cord from the posterior nerve roots (cell bodies in the posterior root ganglia), the fibres form the dorsolateral tract (of Lissauer), and run up or down at the tip of the posterior horn for one or two segments before synapsing with cells of the gelatinous substance.
Through various interneurons in the region of the tip of the posterior horn, the impulses are passed on to the second order neurons whose fibres, mainly from cell bodies in laminae I and V, cross to the opposite side of the cord in front of the central canal and form the anterolateral tract. Although in the lowest part of the cord the fibres from any one segment cross transversely, the crossing takes place more and more obliquely at higher levels so that in the cervical region the decussation may require the height of four or five segments before it is completed. The track is laminated, with fibres subserving impulses from the sacral segments lying most laterally, while those from cervical segments are the most deeply placed (Fig. 7.31). The fibres for crude touch occupy the more medial part of the tract, and have crossed less obliquely than the pain and temperature fibres.
Only about 10% of anterolateral tract fibres pass directly to the thalamus, via the spinal lemniscus of the brainstem (see p. 482). The other 90% of fibres synapse with cells of the brainstem reticular formation, where there may be multiple connections before the onward transmission of impulses to the thalamus; this is the spinoreticulothalamic pathway. Third order neurons then pass from the thalamus to the sensory cortex.
The extent to which the anterolateral tract cells are stimulated to pass on impulses from spinal cord to brain is subject to control and modification by both spinal and supraspinal influences. For example, fibres from mechanoreceptors, apart from passing up in the posterior white columns, give branches that synapse with anterolateral tract cells, and their effect is to inhibit transmission from the tract cells (‘closing the gate’ to nociceptive impulses); hence the effectiveness of rubbing an injured part to reduce the pain. Other inhibitory influences come from supraspinal levels via the reticular formation, so that cerebral and limbic activities such as memory, past experience and emotion can depress or even completely suppress pain, as happens in battle casualties who may feel no pain at the time of severe injury. Certain areas of the nervous system (e.g. the thalamus, limbic system and gelatinous substance) produce their own morphine-like substances (endogenous opioid peptides), such as endorphins and enkephalins, which can inhibit pain by binding to receptors on parts of the pain pathway. Some of these substances may act as modulators of the transmission of pain impulses or may themselves be transmitters. They do not pass the blood–brain barrier and so cannot be given intravenously.
The common tests for pain sensation are by pinprick for superficial pain (spinothalamic) and by pinching or squeezing a fold of skin, muscle or tendon, such as the Achilles tendon, for deep pain (spinoreticulothalamic). Temperature sense can be estimated by touching the skin with a cold metal object and a test-tube of warm water.
In the operation of anterolateral cordotomy for the relief of persistent pain, the lateral part of the anterolateral tract is severed to block upward transmission through the tract. The knife is inserted in front of the line of attachment of the denticulate ligament, so avoiding damage to the corticospinal tract which lies behind this. Because of the obliquity of the crossing of fibres, the cut must be made several segments above the desired level of analgesia. Although cordotomy should theoretically result in permanent pain relief, it rarely lasts for more than a few months (2 years at the most), an
example of the plasticity of the nervous system and the way new pathways can develop. The procedure is rarely performed now on account of advances in analgaesic therapy.
The anterior and posterior spinocerebellar tracts convey unconscious proprioceptive information from cord to cerebellum. Some fibres from posterior root ganglion cells enter the cord through the posterior roots and synapse with the cells of the thoracic nucleus (Clarke's column) at the base of the posterior horn in all the thoracic and first two lumbar segments of the cord. The axons of these cells move to the lateral edge of their own side of the cord as the posterior spinocerebellar tract, which runs up into the medulla to reach the cerebellum by the inferior peduncle. Other incoming fibres synapse with other posterior horn cells in lumbar and sacral segments; these give rise to fibres which mostly cross to the opposite side to form the anterior spinocerebellar tract, at the margin of the cord behind the anterior nerve roots. It takes an unexpectedly long route to the cerebellum, running through the brainstem to the midbrain and doubling back into the superior cerebellar peduncle of the opposite side. From cervical nerves, impulses destined for the cerebellum do not travel by these spinocerebellar tracts (since the thoracic nucleus does not extend above T1 level); they reach the accessory cuneate nucleus in the medulla by the cuneate tract, and thence by the cuneocerebellar tract enter the inferior peduncle.
A number of ill-defined groups of ascending fibres such as spinoreticular, spino-olivary, spinotectal, spinovestibular and spinospinal provide further connections between the cord and brainstem and between different parts of the cord for reflex activities.
Descending tracts
The lateral corticospinal tract is formed from the motor decussation in the lower medulla, and the cortical origin of the fibres has already been described (see p. 481). The tract lies in the lateral white column at a level behind the attachment of the denticulate ligament. Almost all (98%) of the fibres end by synapsing with interneurons which in turn project to the α and γ motor neurons of the anterior horn. (The 2% of fibres that synapse directly with motor neurons are those originating from the giant Betz cells.) About 55% of corticospinal fibres end on cervical and first thoracic anterior horn cells, i.e. more than half of the corticospinal fibres are concerned with the motor supply of the upper limb, leaving 20% for trunk supply and 25% for the lower limb. Because of the medullary decussation, the cerebral cortex of one side controls the muscles of the contralateral side.
The relatively unimportant uncrossed fibres in the medulla continue as the anterior corticospinal tract, adjacent to the anterior median fissure, but it goes no lower than the upper thoracic part of the cord. The fibres eventually cross to the opposite anterior horn.
The most important extrapyramidal tracts are the reticulospinal and the vestibulospinal tracts. The lateral reticulospinal tract arises from the medullary part of the reticular formation and the fibres run down in the lateral white column, largely intermingled with corticospinal fibres. Its influence on anterior horn cells is facilitatory. The medial reticulospinal tract comes from cells in the pontine reticular formation and descends in the anterior white column, to have an inhibitory action on motor neurons. The lateral vestibulospinal tract arises from the lateral vestibular nucleus of the medulla and runs down the cord approximately through the anterior nerve root region. A much smaller medial vestibulospinal tract descends from the medial vestibular nucleus to cervical segments of the cord. The reticuloand vestibulospinal fibres synapse with interneurons which in turn project to the motor
neurons. The lateral vestibulospinal tract primarily affects trunk and limb girdle musculature, and is of great importance for posture and balance; the medial tract coordinates movement of the head.
The hypothalamospinal tract provides a link between the hypothalamus and the thoracolumbar (sympathetic) and sacral (parasympathetic) lateral horn cells. The fibres lie adjacent to the lateral horn.
Clinically it is important to distinguish between upper and lower motor neuron lesions. The lower motor neuron lesion produces a flaccid paralysis of muscles with decreased or absent reflexes. Spastic paralysis with increased relexes, clonus and an extensor plantar response (see p. 18) is regarded as evidence of an upper motor neuron lesion. However, it should be noted that a pure pyramidal lesion (rare, but possible from a lesion confined to the pyramid of the medulla) produces a flaccid paralysis. The reason why pyramidal lesions induce spasticity is that there is concomitant involvement of extrapyramidal pathways as well; the responsiveness of the α motor neurons is altered, possibly because they have been released from the inhibition normally exerted by supraspinal levels. Since most of the pyramidal tract is intermingled with extrapyramidal fibres, most pyramidal lesions present as spasticity.
In the brainstem and spinal cord the motor tracts of each side are quite close together so that a single lesion may easily affect both sides, but in the hemispheres the tracts of the two sides are much farther apart and so unlikely to be damaged together. A single lesion that does affect both sides together is a parasagittal meningioma pressing on the leg areas of both hemispheres.
Blood supply
The spinal cord is supplied by the (single) anterior and (right and left) posterior spinal arteries which descend from the level of the foramen magnum and form three longitudinal channels from which branches enter the cord. They are supplemented at variable levels by anastomoses with a variable number of radicular arteries.
The anterior spinal artery is a midline vessel that lies on the anterior median fissure (Fig. 7.19). It is formed at the foramen magnum by the union of the two anterior spinal branches, each given off by a vertebral artery above the foramen magnum. Although it is usually larger than the posterior spinal arteries and runs the whole length of the cord, the anterior spinal may become so small in places, especially in the thoracic region, that it may be considered absent. It supplies the whole cord anterior to the posterior grey columns, i.e. the lateral grey and white columns and the anterior grey and white columns of both sides.
The posterior spinal artery on each side arises from the posterior inferior cerebellar or vertebral artery above the foramen magnum. It is usually double, forming longitudinal trunks that run through and behind the posterior nerve rootlets for the whole length of the cord. There is some anastomosis between the vessels of the two sides, with rather scanty connections with the anterior spinal artery, except at the lower end of the cord where there are often good anastomoses. The posterior spinal artery supplies the grey and white posterior columns of its own side.
The radicular arteries make highly important contributions to reinforce the longitudinal trunks. At one stage during embryonic development every segment of the cord receives a radicular vessel on both sides; they enter through the intervertebral foramina as spinal arteries to penetrate the meninges
and run along the nerve roots, and are derived from various parent vessels depending on the level: vertebral, costocervical, posterior intercostals, lumbar, and lateral sacral. As fetal growth proceeds, most of the radicular arteries disappear. Those that remain form anastomoses with the anterior and posterior spinal arteries. Their most characteristic feature is their variability in number and position; blood from them may flow up and/or down the cord. On account of the frequently small size of the longitudinal trunks, considerable lengths of the cord may be largely dependent on the radicular supply. The largest of the feeder vessels, the arteria radicularis magna (of Adamkiewicz), usually arises from a lower intercostal or upper lumbar branch of the aorta on the left side. Operations on the vertebral column or adjacent structures (such as aortic aneurysms) that interfere with the parent stem of a major radicular vessel may seriously impair the blood supply to the cord.
The anastomotic connections on the surface of the cord (deep to the pia mater) between the anterior and posterior spinal and radicular vessels provide very small pial arteries that are capable of supplying peripheral areas of the cord. This is important with respect to the lateral corticospinal and anterolateral tracts whose fibres are laminated, with sacral fibres lying nearest to the surface. Interference with the anterior spinal supply may eliminate the function of these tracts, except for the sacral fibres which remain supplied by the pial vessels (‘sacral sparing’).
The spinal veins form loose-knit plexuses in which there are an anterior and a posterior midline longitudinal vein, and on each side a pair of longitudinal veins posterior to the anterior and posterior nerve roots. These veins drain to the internal vertebral venous plexus, and thence via the external vertebral venous plexus to the segmental veins: vertebral in the neck; azygos in the thorax; lumbar in the lumbar region; and lateral sacral in the sacral region. At the foramen magnum they communicate with the veins of the medulla.
Spinal cord injury
In complete transection there is loss of movement and all sensation below the level of the injured segment. The paralysis, which is at first flaccid, becomes spastic after a few weeks, and bladder and rectal sphincter control is lost, although reflex emptying will occur provided the sacral part of the cord is intact. In lesions above T10 segment there is no effective cough because of abdominal and lower intercostal paralysis. In suspected transection, examination for sensation in the perianal skin will establish whether or not there is conduction throughout the length of the cord. Perianal pinprick will also establish whether the anal reflex is intact (see p. 18).
In hemisection (Brown-Séquard syndrome) there is paralysis and loss of touch and kinaesthetic sense below the level of the lesion on the same side (lateral corticospinal tract and posterior column interruption), and loss of pain and temperature sensation on the opposite side (because of interruption of the crossed anterolateral tract).
In the central cord syndrome , commonly due to a crush injury (without transection) following a sudden hyperextension of the cervical spine, there is flaccid (lower motor neuron) paralysis and loss of pain and temperature sensation in the upper limbs (due to anterior horn damage and interruption of the more deeply placed cervical fibres of the anterolateral tracts). The lower limbs may show spasticity if the lumbar fibres of the lateral corticospinal tract are involved (the sacral fibres are more superficial).
In the anterior spinal artery syndrome, the posterior white columns (and therefore touch sensation) remain intact, but most of the rest of the cord below the level of the lesion is affected with loss of all motor and sensory functions, except perhaps for the ‘sacral sparing’ mentioned above.