
- •Textbook Series
- •Contents
- •1 Overview and Definitions
- •Overview
- •General Definitions
- •Glossary
- •List of Symbols
- •Greek Symbols
- •Others
- •Self-assessment Questions
- •Answers
- •2 The Atmosphere
- •Introduction
- •The Physical Properties of Air
- •Static Pressure
- •Temperature
- •Air Density
- •International Standard Atmosphere (ISA)
- •Dynamic Pressure
- •Key Facts
- •Measuring Dynamic Pressure
- •Relationships between Airspeeds
- •Airspeed
- •Errors and Corrections
- •V Speeds
- •Summary
- •Questions
- •Answers
- •3 Basic Aerodynamic Theory
- •The Principle of Continuity
- •Bernoulli’s Theorem
- •Streamlines and the Streamtube
- •Summary
- •Questions
- •Answers
- •4 Subsonic Airflow
- •Aerofoil Terminology
- •Basics about Airflow
- •Two Dimensional Airflow
- •Summary
- •Questions
- •Answers
- •5 Lift
- •Aerodynamic Force Coefficient
- •The Basic Lift Equation
- •Review:
- •The Lift Curve
- •Interpretation of the Lift Curve
- •Density Altitude
- •Aerofoil Section Lift Characteristics
- •Introduction to Drag Characteristics
- •Lift/Drag Ratio
- •Effect of Aircraft Weight on Minimum Flight Speed
- •Condition of the Surface
- •Flight at High Lift Conditions
- •Three Dimensional Airflow
- •Wing Terminology
- •Wing Tip Vortices
- •Wake Turbulence: (Ref: AIC P 072/2010)
- •Ground Effect
- •Conclusion
- •Summary
- •Answers from page 77
- •Answers from page 78
- •Questions
- •Answers
- •6 Drag
- •Introduction
- •Parasite Drag
- •Induced Drag
- •Methods of Reducing Induced Drag
- •Effect of Lift on Parasite Drag
- •Aeroplane Total Drag
- •The Effect of Aircraft Gross Weight on Total Drag
- •The Effect of Altitude on Total Drag
- •The Effect of Configuration on Total Drag
- •Speed Stability
- •Power Required (Introduction)
- •Summary
- •Questions
- •Annex C
- •Answers
- •7 Stalling
- •Introduction
- •Cause of the Stall
- •The Lift Curve
- •Stall Recovery
- •Aircraft Behaviour Close to the Stall
- •Use of Flight Controls Close to the Stall
- •Stall Recognition
- •Stall Speed
- •Stall Warning
- •Artificial Stall Warning Devices
- •Basic Stall Requirements (EASA and FAR)
- •Wing Design Characteristics
- •The Effect of Aerofoil Section
- •The Effect of Wing Planform
- •Key Facts 1
- •Super Stall (Deep Stall)
- •Factors that Affect Stall Speed
- •1g Stall Speed
- •Effect of Weight Change on Stall Speed
- •Composition and Resolution of Forces
- •Using Trigonometry to Resolve Forces
- •Lift Increase in a Level Turn
- •Effect of Load Factor on Stall Speed
- •Effect of High Lift Devices on Stall Speed
- •Effect of CG Position on Stall Speed
- •Effect of Landing Gear on the Stall Speed
- •Effect of Engine Power on Stall Speed
- •Effect of Mach Number (Compressibility) on Stall Speed
- •Effect of Wing Contamination on Stall Speed
- •Warning to the Pilot of Icing-induced Stalls
- •Stabilizer Stall Due to Ice
- •Effect of Heavy Rain on Stall Speed
- •Stall and Recovery Characteristics of Canards
- •Spinning
- •Primary Causes of a Spin
- •Phases of a Spin
- •The Effect of Mass and Balance on Spins
- •Spin Recovery
- •Special Phenomena of Stall
- •High Speed Buffet (Shock Stall)
- •Answers to Questions on Page 173
- •Key Facts 2
- •Questions
- •Key Facts 1 (Completed)
- •Key Facts 2 (Completed)
- •Answers
- •8 High Lift Devices
- •Purpose of High Lift Devices
- •Take-off and Landing Speeds
- •Augmentation
- •Flaps
- •Trailing Edge Flaps
- •Plain Flap
- •Split Flap
- •Slotted and Multiple Slotted Flaps
- •The Fowler Flap
- •Comparison of Trailing Edge Flaps
- •and Stalling Angle
- •Drag
- •Lift / Drag Ratio
- •Pitching Moment
- •Centre of Pressure Movement
- •Change of Downwash
- •Overall Pitch Change
- •Aircraft Attitude with Flaps Lowered
- •Leading Edge High Lift Devices
- •Leading Edge Flaps
- •Effect of Leading Edge Flaps on Lift
- •Leading Edge Slots
- •Leading Edge Slat
- •Automatic Slots
- •Disadvantages of the Slot
- •Drag and Pitching Moment of Leading Edge Devices
- •Trailing Edge Plus Leading Edge Devices
- •Sequence of Operation
- •Asymmetry of High Lift Devices
- •Flap Load Relief System
- •Choice of Flap Setting for Take-off, Climb and Landing
- •Management of High Lift Devices
- •Flap Extension Prior to Landing
- •Questions
- •Annexes
- •Answers
- •9 Airframe Contamination
- •Introduction
- •Types of Contamination
- •Effect of Frost and Ice on the Aircraft
- •Effect on Instruments
- •Effect on Controls
- •Water Contamination
- •Airframe Aging
- •Questions
- •Answers
- •10 Stability and Control
- •Introduction
- •Static Stability
- •Aeroplane Reference Axes
- •Static Longitudinal Stability
- •Neutral Point
- •Static Margin
- •Trim and Controllability
- •Key Facts 1
- •Graphic Presentation of Static Longitudinal Stability
- •Contribution of the Component Surfaces
- •Power-off Stability
- •Effect of CG Position
- •Power Effects
- •High Lift Devices
- •Control Force Stability
- •Manoeuvre Stability
- •Stick Force Per ‘g’
- •Tailoring Control Forces
- •Longitudinal Control
- •Manoeuvring Control Requirement
- •Take-off Control Requirement
- •Landing Control Requirement
- •Dynamic Stability
- •Longitudinal Dynamic Stability
- •Long Period Oscillation (Phugoid)
- •Short Period Oscillation
- •Directional Stability and Control
- •Sideslip Angle
- •Static Directional Stability
- •Contribution of the Aeroplane Components.
- •Lateral Stability and Control
- •Static Lateral Stability
- •Contribution of the Aeroplane Components
- •Lateral Dynamic Effects
- •Spiral Divergence
- •Dutch Roll
- •Pilot Induced Oscillation (PIO)
- •High Mach Numbers
- •Mach Trim
- •Key Facts 2
- •Summary
- •Questions
- •Key Facts 1 (Completed)
- •Key Facts 2 (Completed)
- •Answers
- •11 Controls
- •Introduction
- •Hinge Moments
- •Control Balancing
- •Mass Balance
- •Longitudinal Control
- •Lateral Control
- •Speed Brakes
- •Directional Control
- •Secondary Effects of Controls
- •Trimming
- •Questions
- •Answers
- •12 Flight Mechanics
- •Introduction
- •Straight Horizontal Steady Flight
- •Tailplane and Elevator
- •Balance of Forces
- •Straight Steady Climb
- •Climb Angle
- •Effect of Weight, Altitude and Temperature.
- •Power-on Descent
- •Emergency Descent
- •Glide
- •Rate of Descent in the Glide
- •Turning
- •Flight with Asymmetric Thrust
- •Summary of Minimum Control Speeds
- •Questions
- •Answers
- •13 High Speed Flight
- •Introduction
- •Speed of Sound
- •Mach Number
- •Effect on Mach Number of Climbing at a Constant IAS
- •Variation of TAS with Altitude at a Constant Mach Number
- •Influence of Temperature on Mach Number at a Constant Flight Level and IAS
- •Subdivisions of Aerodynamic Flow
- •Propagation of Pressure Waves
- •Normal Shock Waves
- •Critical Mach Number
- •Pressure Distribution at Transonic Mach Numbers
- •Properties of a Normal Shock Wave
- •Oblique Shock Waves
- •Effects of Shock Wave Formation
- •Buffet
- •Factors Which Affect the Buffet Boundaries
- •The Buffet Margin
- •Use of the Buffet Onset Chart
- •Delaying or Reducing the Effects of Compressibility
- •Aerodynamic Heating
- •Mach Angle
- •Mach Cone
- •Area (Zone) of Influence
- •Bow Wave
- •Expansion Waves
- •Sonic Bang
- •Methods of Improving Control at Transonic Speeds
- •Questions
- •Answers
- •14 Limitations
- •Operating Limit Speeds
- •Loads and Safety Factors
- •Loads on the Structure
- •Load Factor
- •Boundary
- •Design Manoeuvring Speed, V
- •Effect of Altitude on V
- •Effect of Aircraft Weight on V
- •Design Cruising Speed V
- •Design Dive Speed V
- •Negative Load Factors
- •The Negative Stall
- •Manoeuvre Boundaries
- •Operational Speed Limits
- •Gust Loads
- •Effect of a Vertical Gust on the Load Factor
- •Effect of the Gust on Stalling
- •Operational Rough-air Speed (V
- •Landing Gear Speed Limitations
- •Flap Speed Limit
- •Aeroelasticity (Aeroelastic Coupling)
- •Flutter
- •Control Surface Flutter
- •Aileron Reversal
- •Questions
- •Answers
- •15 Windshear
- •Introduction (Ref: AIC 84/2008)
- •Microburst
- •Windshear Encounter during Approach
- •Effects of Windshear
- •“Typical” Recovery from Windshear
- •Windshear Reporting
- •Visual Clues
- •Conclusions
- •Questions
- •Answers
- •16 Propellers
- •Introduction
- •Definitions
- •Aerodynamic Forces on the Propeller
- •Thrust
- •Centrifugal Twisting Moment (CTM)
- •Propeller Efficiency
- •Variable Pitch Propellers
- •Power Absorption
- •Moments and Forces Generated by a Propeller
- •Effect of Atmospheric Conditions
- •Questions
- •Answers
- •17 Revision Questions
- •Questions
- •Answers
- •Explanations to Specimen Questions
- •Specimen Examination Paper
- •Answers to Specimen Exam Paper
- •Explanations to Specimen Exam Paper
- •18 Index
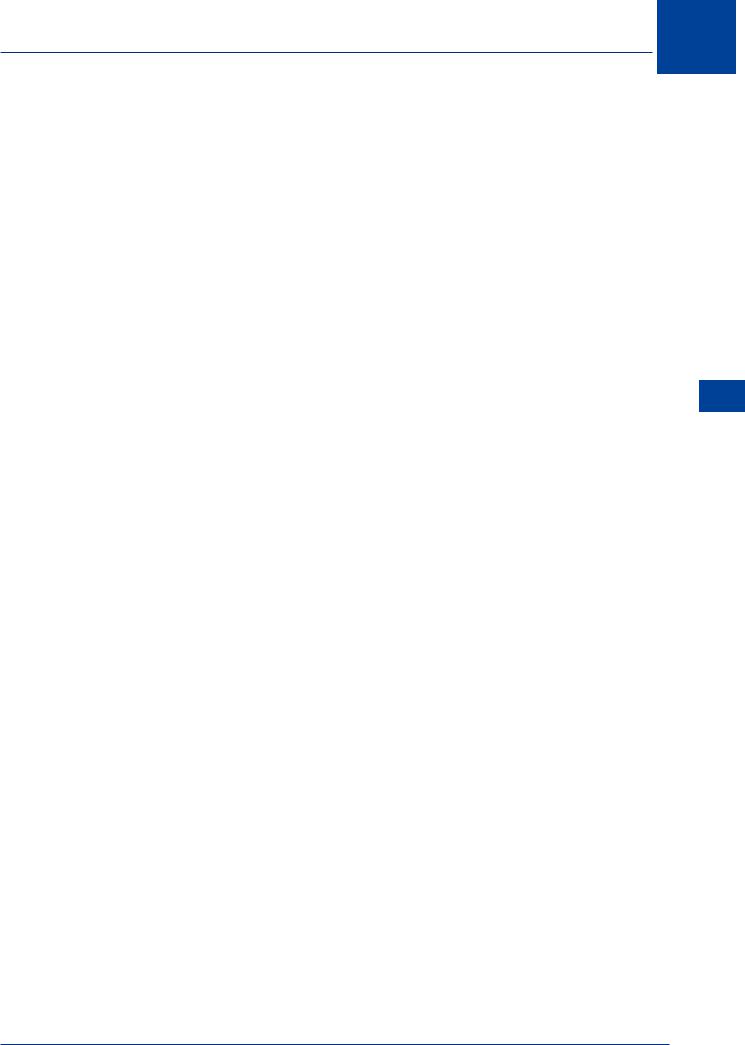
Stability and Control 10
Lateral Stability and Control
The static lateral stability of an aeroplane involves consideration of rolling moments due to sideslip. If an aeroplane has favourable rolling moment due to a sideslip, a lateral displacement from wing level flight produces a sideslip, and the sideslip creates a rolling moment tending to return the aeroplane to wing level flight. By this action, static lateral stability will be evident. Of course, a sideslip will produce yawing moments depending on the nature of the static directional stability, but the consideration of static lateral stability will involve only the relationship of rolling moments and sideslip.
Definitions
The axis system of an aeroplane defines a positive rolling, L, as a moment about the longitudinal axis which tends to rotate the right wing down. As in other aerodynamic considerations, it is convenient to consider rolling moments in the coefficient form so that lateral stability can be evaluated independent of weight, altitude, speeds, etc. The rolling moment, L, is defined in the coefficient form by the following equation:
L = Cl Q S b
or
Cl = |
L |
Q S b |
where:
L = rolling moment (positive to right)
Q = dynamic pressure
S = wing area
b = wingspan
Cl = rolling moment coefficient (positive to the right)
The angle of sideslip, β,has been defined previously as the angle between the aeroplane centre line and the relative wind and is positive when the relative wind is to the right of the centre line.
Stability and Control 10
301
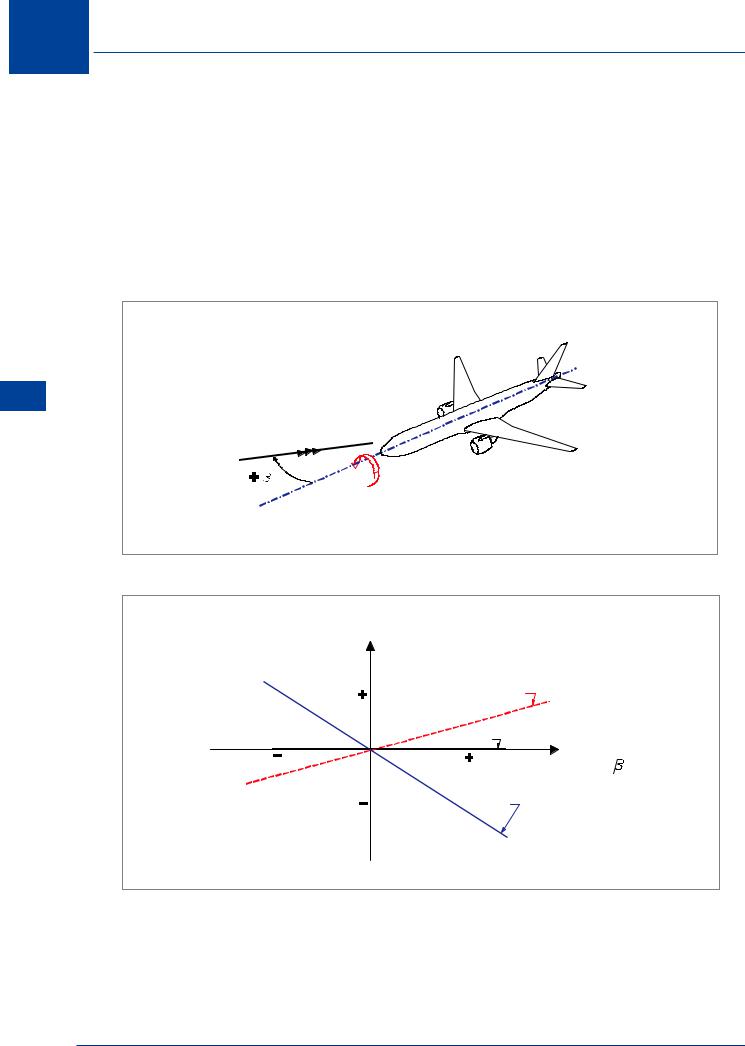
10 Stability and Control
Static Lateral Stability
Control and Stability 10
Static lateral stability can be illustrated by a graph of rolling moment coefficient, Cl, versus sideslip angle, β, such as shown in Figure 10.67. When the aeroplane is subject to a positive sideslip angle, lateral stability will be evident if a negative rolling moment coefficient results. Thus, when the relative airflow comes from the right (+β), a rolling moment to the left (-Cl) should be created which tends to roll the aeroplane to the left. Lateral stability will exist when the curve of Cl versus β has a negative slope and the degree of stability will be a function of the slope of this curve. If the slope of the curve is zero, neutral lateral stability exists; if the slope is positive, lateral instability is present.
RELATIVE AIRFLOW
l, ROLLING MOMENT
Figure 10.66
ROLLING MOMENT COEFFICIENT |
Cl |
UNSTABLE |
NEUTRAL |
SIDESLIP ANGLE, |
STABLE |
Figure 10.67
302
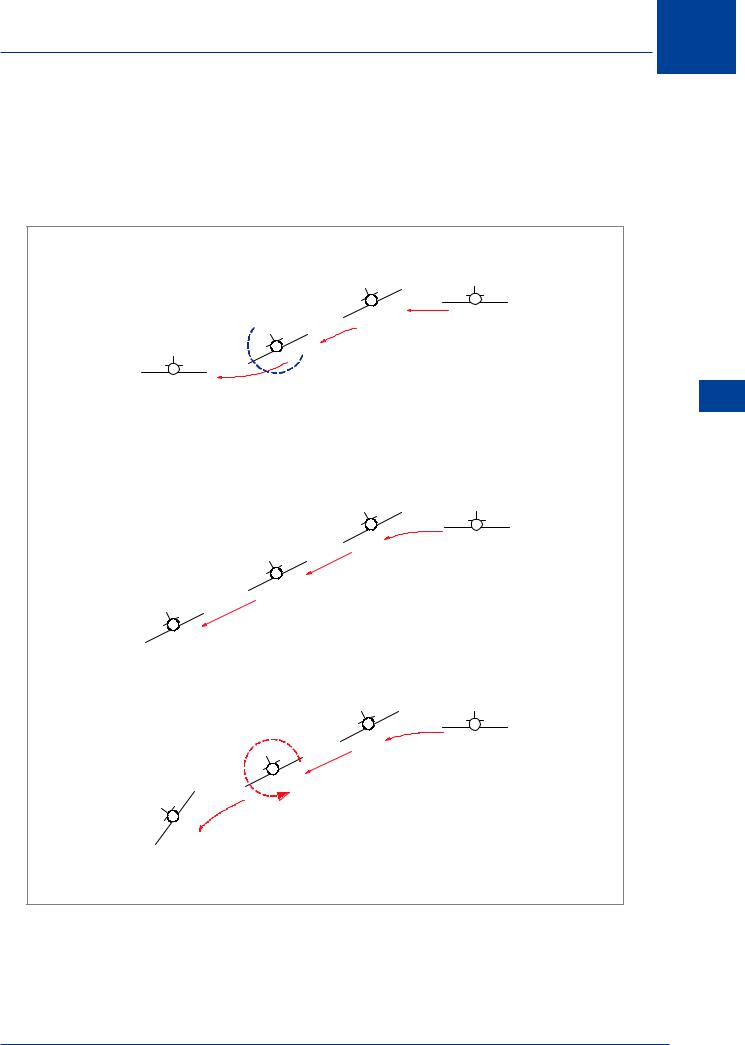
Stability and Control 10
It is desirable to have static lateral stability (favourable roll due to sideslip), Figure 10.68. However, the required magnitude of lateral stability is determined by many factors. Excessive roll due to sideslip complicates crosswind take-off and landing and may lead to undesirable oscillatory coupling with the directional motion of the aeroplane. In addition, high lateral stability may combine with adverse yaw to hinder rolling performance. Generally, good handling qualities are obtained with a relatively light, or weak positive, lateral stability.
STABLE ROLL DUE
TO SIDESLIP
Stability and Control 10
NEUTRAL
UNSTABLE ROLL
DUE TO SIDESLIP
Figure 10.68 Static lateral stability
303
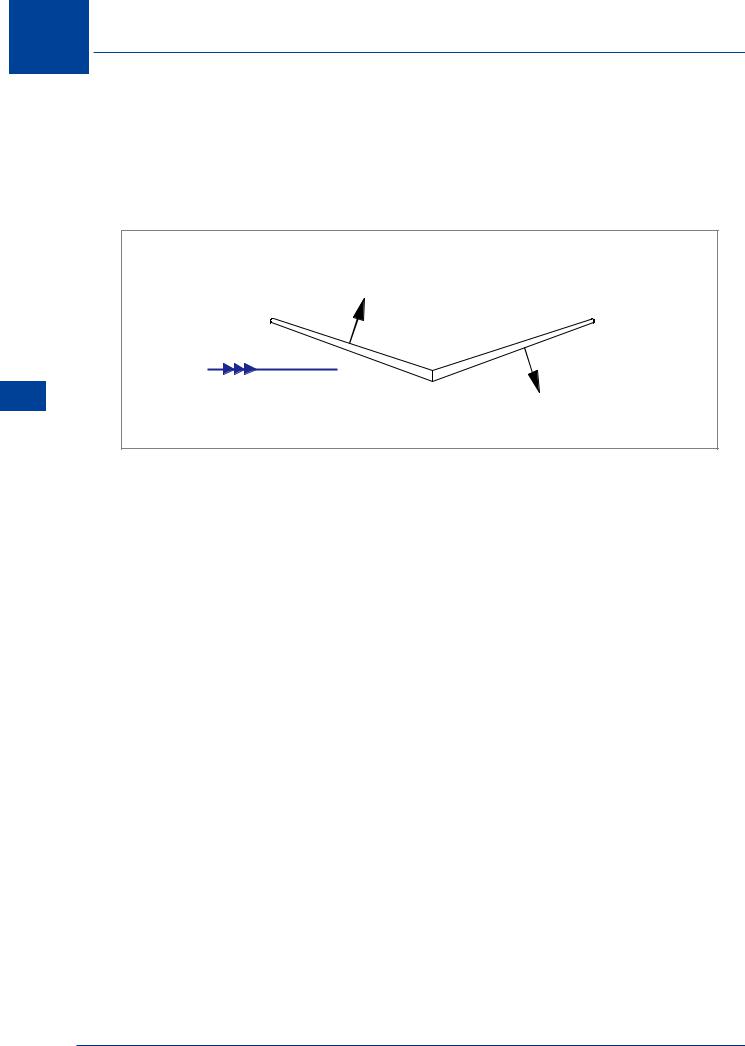
10 Stability and Control
Contribution of the Aeroplane Components
Control and Stability 10
In order to appreciate the development of lateral stability in an aeroplane, each of the components which contribute must be inspected. There will be interference between the components, which will alter the contribution to stability of each component on the aeroplane.
EFFECTIVE INCREASE IN
LIFT DUE TO SIDESLIP
EFFECTIVE DECREASE IN
LIFT DUE TO SIDESLIP
Figure 10.69 Geometric dihedral
Wing
The principal surface contributing to the lateral stability of an aeroplane is the wing. The effect of *geometric dihedral is a powerful contribution to lateral stability.
As shown in Figure 10.69, a wing with geometric dihedral will develop stable rolling moments with sideslip. If the relative wind comes from the side, the wing into the wind is subject to an increase in angle of attack and develops an increase in lift. The wing away from the wind is subject to a decrease in angle of attack and develops a decrease in lift. The changes in lift gives a rolling moment tending to raise the into-wind wing, hence geometric dihedral contributes a stable roll due to sideslip.
Since geometric dihedral is so powerful in producing lateral stability it is taken as a common denominator of the lateral stability contribution of all other components. Generally, the contribution of wing position, flaps, power, etc., is expressed as “DIHEDRAL EFFECT”.
*Geometric Dihedral: The angle between the plane of each wing and the horizontal, when the aircraft is unbanked and level; positive when the wing lies above the horizontal, as in Figure 10.69. Negative geometric dihedral is used on some aircraft, and is known as anhedral.
304
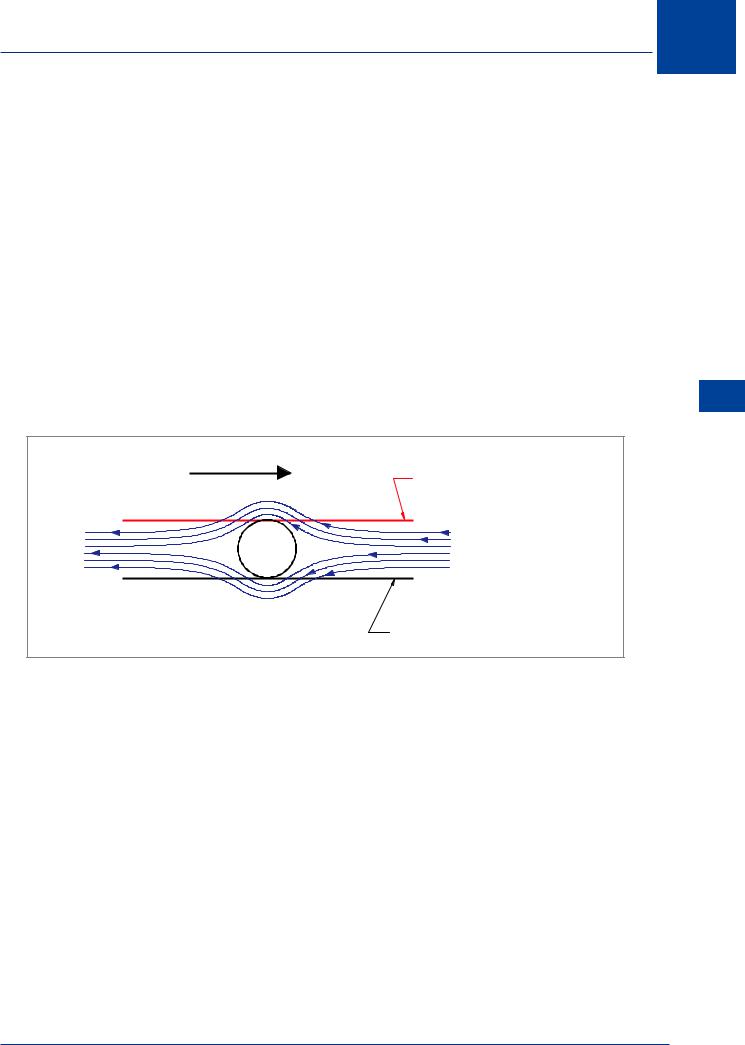
Stability and Control
Wing Position
The contribution of the fuselage alone is usually quite small; depending on the location of the resultant aerodynamic side force on the fuselage.
However, the effect of the wing - fuselage - tail combination is significant since the vertical placement of the wing on the fuselage can greatly affect the combination. A wing located at the mid wing position will generally exhibit a “dihedral effect” no different from that of the wing alone.
Figure 10.70 illustrates the effect of wing position on static lateral stability.
•A low wing position gives an unstable contribution. The direction of relative airflow decreases the effective angle of attack of the wing into wind and increases the effective angle of attack of the wing out of wind - tending to increase the rolling moment.
•A high wing location gives a stable contribution. The direction of relative airflow increases the effective angle of attack of the wing into wind and decreases the effective angle of attack of the wing out of wind, tending to decrease the rolling moment.
SIDESLIP
HIGH WING POSITION
LOW WING POSITION
Figure 10.70 Wing - fuselage interference effect
The magnitude of “dihedral effect” contributed by the vertical position of the wing is large and may require a noticeable dihedral angle for the low wing configuration. A high wing position, on the other hand, usually requires no geometric dihedral at all.
10
Stability and Control 10
305
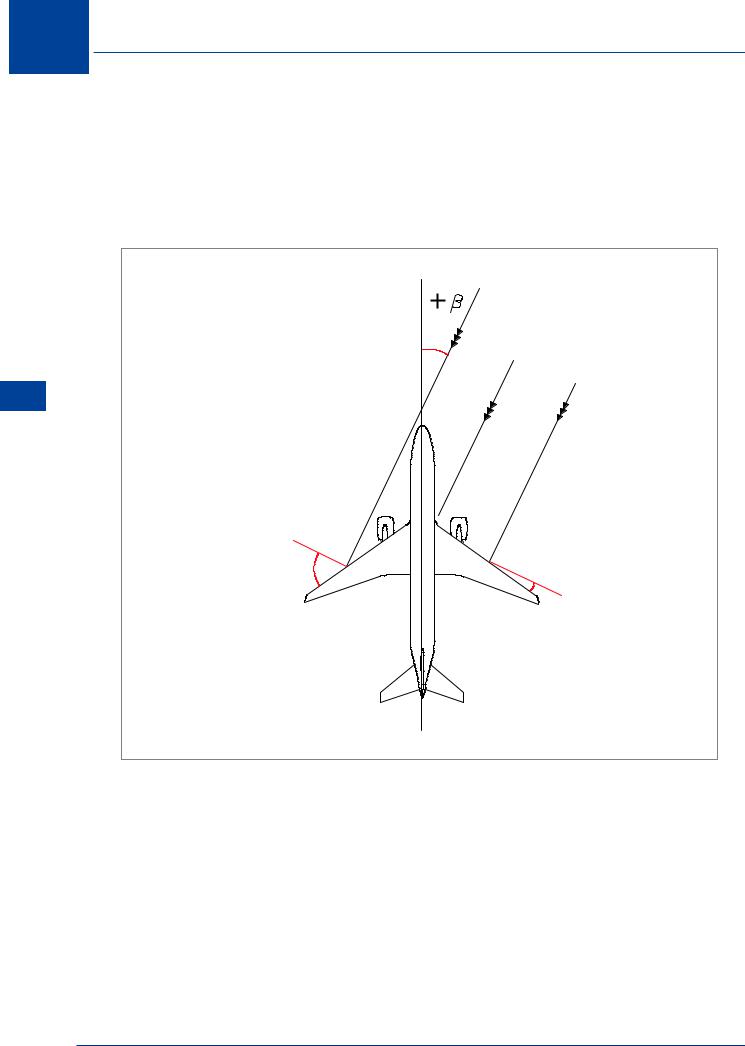
10 Stability and Control
Sweepback
The contribution of sweepback to “dihedral effect” is important because of the nature of the contribution. As shown in Figure 10.71 and Figure 10.72, if the wing is at a positive lift coefficient, the wing into the wind has less sweep and an increase in lift, and the wing out of the wind has more sweep and a decrease in lift; a negative rolling moment will be generated, tending to roll the wings towards level. In this manner the swept-back wing contributes a positive “dihedral effect”. (A swept-forward wing would give a negative dihedral effect).
Control and Stability 10
INCREASED
EFFECTIVE
SWEEP
DECREASED
EFFECTIVE
SWEEP
Figure 10.71 The effect of sweepback
The contribution of sweepback to “dihedral effect” is proportional to the wing lift coefficient as well as the angle of sweepback. Because high speed flight requires a large amount of sweepback, an excessively high “dihedral effect” will be present at low speeds (high CL). An aircraft with a swept-back wing requires less geometric dihedral than a straight wing.
306
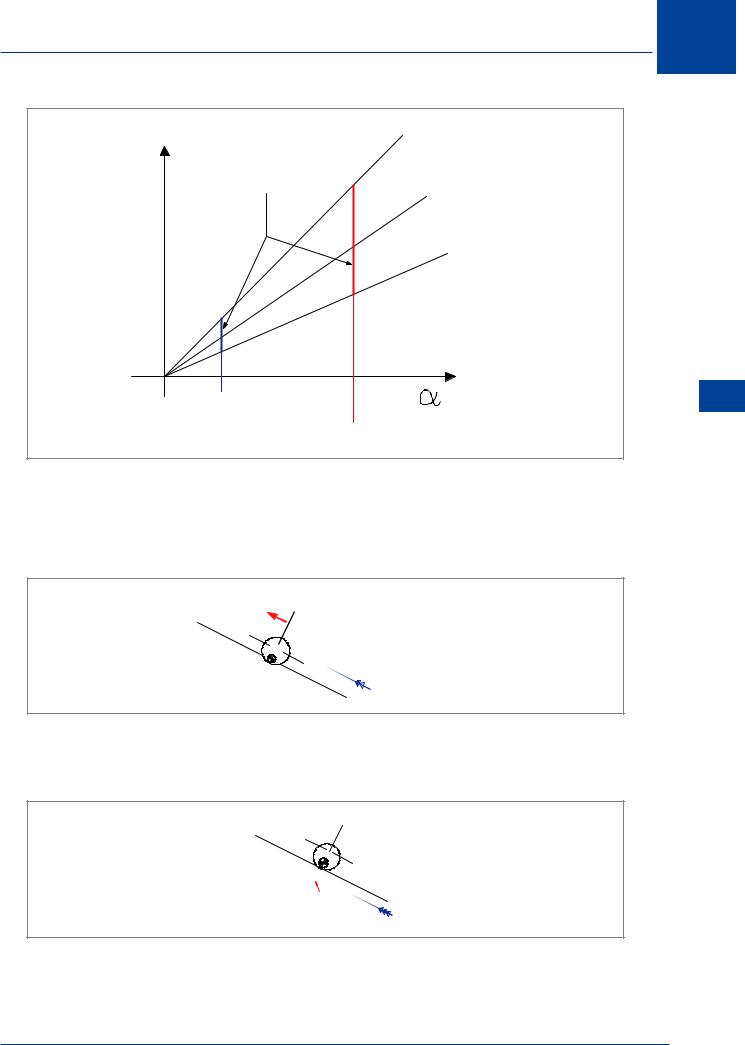
Stability and Control 10
|
|
LEADING WING |
|
|
IN SIDESLIP |
CL |
DIFFERENCE IN |
|
LIFT ON THE |
|
|
TWO WINGS |
ZERO |
|
|
|
SIDESLIP |
|
|
TRAILING WING |
|
|
IN SIDESLIP |
0 |
HIGH |
|
|
|
|
|
SPEED |
|
|
|
LOW |
|
|
SPEED |
Figure 10.72 Effect of speed on ‘Dihedral Effect’ of swept wing
The fin can provide a small “dihedral effect” contribution, Figure 10.73. If the fin is large, the side force produced by sideslip may produce a rolling moment as well as the important yawing moment contribution. The fin contribution to purely lateral static stability is usually very small.
SMALL STABILIZING
ROLLING MOMENT IN
SIDESLIP
RELATIVE AIRFLOW
Figure 10.73 Fin contribution
The ventral fin, being below the aircraft CG, has a negative influence on lateral static stability, as illustrated in Figure 10.74.
SMALL DESTABILIZING
ROLLING MOMENT
IN SIDESLIP
VENTRAL
FIN
RELATIVE AIRFLOW
Figure 10.74 Ventral fin contribution
Generally, the “dihedral effect” should not be too great since high roll due to sideslip can create certain problems.
Stability and Control 10
307
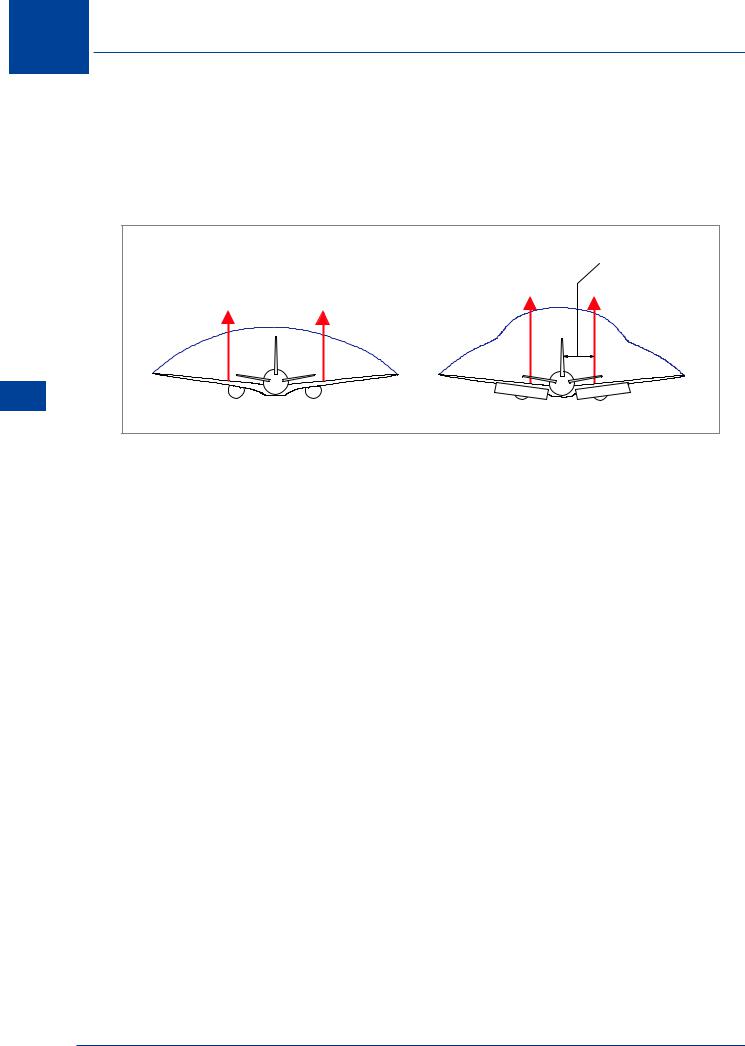
10 Stability and Control
Control and Stability 10
Excessive “dihedral effect” can lead to “Dutch roll” difficult rudder coordination in rolling manoeuvres, or place extreme demands for lateral control power during crosswind take-off and landing. If the aeroplane demonstrates satisfactory “dihedral effect” during cruise, certain exceptions can be tolerated when the aeroplane is in the take-off and landing configuration. Since the effects of flaps and power are destabilizing and reduce the “dihedral effect”, a certain amount of negative “dihedral effect” may be possible due to these sources.
REDUCED ARM |
Figure 10.75 Partial span flaps reduce lateral stability
The deflection of flaps causes the inboard sections of the wing to become relatively more effective and these sections have a small spanwise moment arm, Figure 10.75. Therefore, the changes in wing lift due to sideslip occur closer inboard and the dihedral effect is reduced.
The effect of power on “dihedral effect” is negligible for the jet aeroplane but considerable for the propeller driven aeroplane. The propeller slipstream at high power and low airspeed makes the inboard wing sections much more effective and reduces the dihedral effect.
The reduction in “dihedral effect” is most critical when the flap and power effects are combined, e.g. the propeller driven aeroplane in a power-on approach.
With certain exceptions during the conditions of landing and take-off, the “dihedral effect” or lateral stability should be positive but light. The problems created by excessive “dihedral effect” are considerable and difficult to contend with. Lateral stability will be evident to a pilot by stick forces and displacements required to maintain sideslip. Positive stick force stability will be evident by stick forces required in the direction of the controlled sideslip.
Conclusion
The designer is faced with a dilemma. An aircraft is given sweepback to increase the speed at which it can operate, but a by-product of sweepback is static lateral stability. A swept-back wing requires much less geometric dihedral than a straight wing. If a requirement also exists for the wing to be mounted on top of the fuselage, an additional “dihedral effect”is present. A high mounted and swept-back wing would give excessive “dihedral effect”, so anhedral is used to reduce “dihedral effect” to the required level.
308