
- •Textbook Series
- •Contents
- •1 Overview and Definitions
- •Overview
- •General Definitions
- •Glossary
- •List of Symbols
- •Greek Symbols
- •Others
- •Self-assessment Questions
- •Answers
- •2 The Atmosphere
- •Introduction
- •The Physical Properties of Air
- •Static Pressure
- •Temperature
- •Air Density
- •International Standard Atmosphere (ISA)
- •Dynamic Pressure
- •Key Facts
- •Measuring Dynamic Pressure
- •Relationships between Airspeeds
- •Airspeed
- •Errors and Corrections
- •V Speeds
- •Summary
- •Questions
- •Answers
- •3 Basic Aerodynamic Theory
- •The Principle of Continuity
- •Bernoulli’s Theorem
- •Streamlines and the Streamtube
- •Summary
- •Questions
- •Answers
- •4 Subsonic Airflow
- •Aerofoil Terminology
- •Basics about Airflow
- •Two Dimensional Airflow
- •Summary
- •Questions
- •Answers
- •5 Lift
- •Aerodynamic Force Coefficient
- •The Basic Lift Equation
- •Review:
- •The Lift Curve
- •Interpretation of the Lift Curve
- •Density Altitude
- •Aerofoil Section Lift Characteristics
- •Introduction to Drag Characteristics
- •Lift/Drag Ratio
- •Effect of Aircraft Weight on Minimum Flight Speed
- •Condition of the Surface
- •Flight at High Lift Conditions
- •Three Dimensional Airflow
- •Wing Terminology
- •Wing Tip Vortices
- •Wake Turbulence: (Ref: AIC P 072/2010)
- •Ground Effect
- •Conclusion
- •Summary
- •Answers from page 77
- •Answers from page 78
- •Questions
- •Answers
- •6 Drag
- •Introduction
- •Parasite Drag
- •Induced Drag
- •Methods of Reducing Induced Drag
- •Effect of Lift on Parasite Drag
- •Aeroplane Total Drag
- •The Effect of Aircraft Gross Weight on Total Drag
- •The Effect of Altitude on Total Drag
- •The Effect of Configuration on Total Drag
- •Speed Stability
- •Power Required (Introduction)
- •Summary
- •Questions
- •Annex C
- •Answers
- •7 Stalling
- •Introduction
- •Cause of the Stall
- •The Lift Curve
- •Stall Recovery
- •Aircraft Behaviour Close to the Stall
- •Use of Flight Controls Close to the Stall
- •Stall Recognition
- •Stall Speed
- •Stall Warning
- •Artificial Stall Warning Devices
- •Basic Stall Requirements (EASA and FAR)
- •Wing Design Characteristics
- •The Effect of Aerofoil Section
- •The Effect of Wing Planform
- •Key Facts 1
- •Super Stall (Deep Stall)
- •Factors that Affect Stall Speed
- •1g Stall Speed
- •Effect of Weight Change on Stall Speed
- •Composition and Resolution of Forces
- •Using Trigonometry to Resolve Forces
- •Lift Increase in a Level Turn
- •Effect of Load Factor on Stall Speed
- •Effect of High Lift Devices on Stall Speed
- •Effect of CG Position on Stall Speed
- •Effect of Landing Gear on the Stall Speed
- •Effect of Engine Power on Stall Speed
- •Effect of Mach Number (Compressibility) on Stall Speed
- •Effect of Wing Contamination on Stall Speed
- •Warning to the Pilot of Icing-induced Stalls
- •Stabilizer Stall Due to Ice
- •Effect of Heavy Rain on Stall Speed
- •Stall and Recovery Characteristics of Canards
- •Spinning
- •Primary Causes of a Spin
- •Phases of a Spin
- •The Effect of Mass and Balance on Spins
- •Spin Recovery
- •Special Phenomena of Stall
- •High Speed Buffet (Shock Stall)
- •Answers to Questions on Page 173
- •Key Facts 2
- •Questions
- •Key Facts 1 (Completed)
- •Key Facts 2 (Completed)
- •Answers
- •8 High Lift Devices
- •Purpose of High Lift Devices
- •Take-off and Landing Speeds
- •Augmentation
- •Flaps
- •Trailing Edge Flaps
- •Plain Flap
- •Split Flap
- •Slotted and Multiple Slotted Flaps
- •The Fowler Flap
- •Comparison of Trailing Edge Flaps
- •and Stalling Angle
- •Drag
- •Lift / Drag Ratio
- •Pitching Moment
- •Centre of Pressure Movement
- •Change of Downwash
- •Overall Pitch Change
- •Aircraft Attitude with Flaps Lowered
- •Leading Edge High Lift Devices
- •Leading Edge Flaps
- •Effect of Leading Edge Flaps on Lift
- •Leading Edge Slots
- •Leading Edge Slat
- •Automatic Slots
- •Disadvantages of the Slot
- •Drag and Pitching Moment of Leading Edge Devices
- •Trailing Edge Plus Leading Edge Devices
- •Sequence of Operation
- •Asymmetry of High Lift Devices
- •Flap Load Relief System
- •Choice of Flap Setting for Take-off, Climb and Landing
- •Management of High Lift Devices
- •Flap Extension Prior to Landing
- •Questions
- •Annexes
- •Answers
- •9 Airframe Contamination
- •Introduction
- •Types of Contamination
- •Effect of Frost and Ice on the Aircraft
- •Effect on Instruments
- •Effect on Controls
- •Water Contamination
- •Airframe Aging
- •Questions
- •Answers
- •10 Stability and Control
- •Introduction
- •Static Stability
- •Aeroplane Reference Axes
- •Static Longitudinal Stability
- •Neutral Point
- •Static Margin
- •Trim and Controllability
- •Key Facts 1
- •Graphic Presentation of Static Longitudinal Stability
- •Contribution of the Component Surfaces
- •Power-off Stability
- •Effect of CG Position
- •Power Effects
- •High Lift Devices
- •Control Force Stability
- •Manoeuvre Stability
- •Stick Force Per ‘g’
- •Tailoring Control Forces
- •Longitudinal Control
- •Manoeuvring Control Requirement
- •Take-off Control Requirement
- •Landing Control Requirement
- •Dynamic Stability
- •Longitudinal Dynamic Stability
- •Long Period Oscillation (Phugoid)
- •Short Period Oscillation
- •Directional Stability and Control
- •Sideslip Angle
- •Static Directional Stability
- •Contribution of the Aeroplane Components.
- •Lateral Stability and Control
- •Static Lateral Stability
- •Contribution of the Aeroplane Components
- •Lateral Dynamic Effects
- •Spiral Divergence
- •Dutch Roll
- •Pilot Induced Oscillation (PIO)
- •High Mach Numbers
- •Mach Trim
- •Key Facts 2
- •Summary
- •Questions
- •Key Facts 1 (Completed)
- •Key Facts 2 (Completed)
- •Answers
- •11 Controls
- •Introduction
- •Hinge Moments
- •Control Balancing
- •Mass Balance
- •Longitudinal Control
- •Lateral Control
- •Speed Brakes
- •Directional Control
- •Secondary Effects of Controls
- •Trimming
- •Questions
- •Answers
- •12 Flight Mechanics
- •Introduction
- •Straight Horizontal Steady Flight
- •Tailplane and Elevator
- •Balance of Forces
- •Straight Steady Climb
- •Climb Angle
- •Effect of Weight, Altitude and Temperature.
- •Power-on Descent
- •Emergency Descent
- •Glide
- •Rate of Descent in the Glide
- •Turning
- •Flight with Asymmetric Thrust
- •Summary of Minimum Control Speeds
- •Questions
- •Answers
- •13 High Speed Flight
- •Introduction
- •Speed of Sound
- •Mach Number
- •Effect on Mach Number of Climbing at a Constant IAS
- •Variation of TAS with Altitude at a Constant Mach Number
- •Influence of Temperature on Mach Number at a Constant Flight Level and IAS
- •Subdivisions of Aerodynamic Flow
- •Propagation of Pressure Waves
- •Normal Shock Waves
- •Critical Mach Number
- •Pressure Distribution at Transonic Mach Numbers
- •Properties of a Normal Shock Wave
- •Oblique Shock Waves
- •Effects of Shock Wave Formation
- •Buffet
- •Factors Which Affect the Buffet Boundaries
- •The Buffet Margin
- •Use of the Buffet Onset Chart
- •Delaying or Reducing the Effects of Compressibility
- •Aerodynamic Heating
- •Mach Angle
- •Mach Cone
- •Area (Zone) of Influence
- •Bow Wave
- •Expansion Waves
- •Sonic Bang
- •Methods of Improving Control at Transonic Speeds
- •Questions
- •Answers
- •14 Limitations
- •Operating Limit Speeds
- •Loads and Safety Factors
- •Loads on the Structure
- •Load Factor
- •Boundary
- •Design Manoeuvring Speed, V
- •Effect of Altitude on V
- •Effect of Aircraft Weight on V
- •Design Cruising Speed V
- •Design Dive Speed V
- •Negative Load Factors
- •The Negative Stall
- •Manoeuvre Boundaries
- •Operational Speed Limits
- •Gust Loads
- •Effect of a Vertical Gust on the Load Factor
- •Effect of the Gust on Stalling
- •Operational Rough-air Speed (V
- •Landing Gear Speed Limitations
- •Flap Speed Limit
- •Aeroelasticity (Aeroelastic Coupling)
- •Flutter
- •Control Surface Flutter
- •Aileron Reversal
- •Questions
- •Answers
- •15 Windshear
- •Introduction (Ref: AIC 84/2008)
- •Microburst
- •Windshear Encounter during Approach
- •Effects of Windshear
- •“Typical” Recovery from Windshear
- •Windshear Reporting
- •Visual Clues
- •Conclusions
- •Questions
- •Answers
- •16 Propellers
- •Introduction
- •Definitions
- •Aerodynamic Forces on the Propeller
- •Thrust
- •Centrifugal Twisting Moment (CTM)
- •Propeller Efficiency
- •Variable Pitch Propellers
- •Power Absorption
- •Moments and Forces Generated by a Propeller
- •Effect of Atmospheric Conditions
- •Questions
- •Answers
- •17 Revision Questions
- •Questions
- •Answers
- •Explanations to Specimen Questions
- •Specimen Examination Paper
- •Answers to Specimen Exam Paper
- •Explanations to Specimen Exam Paper
- •18 Index
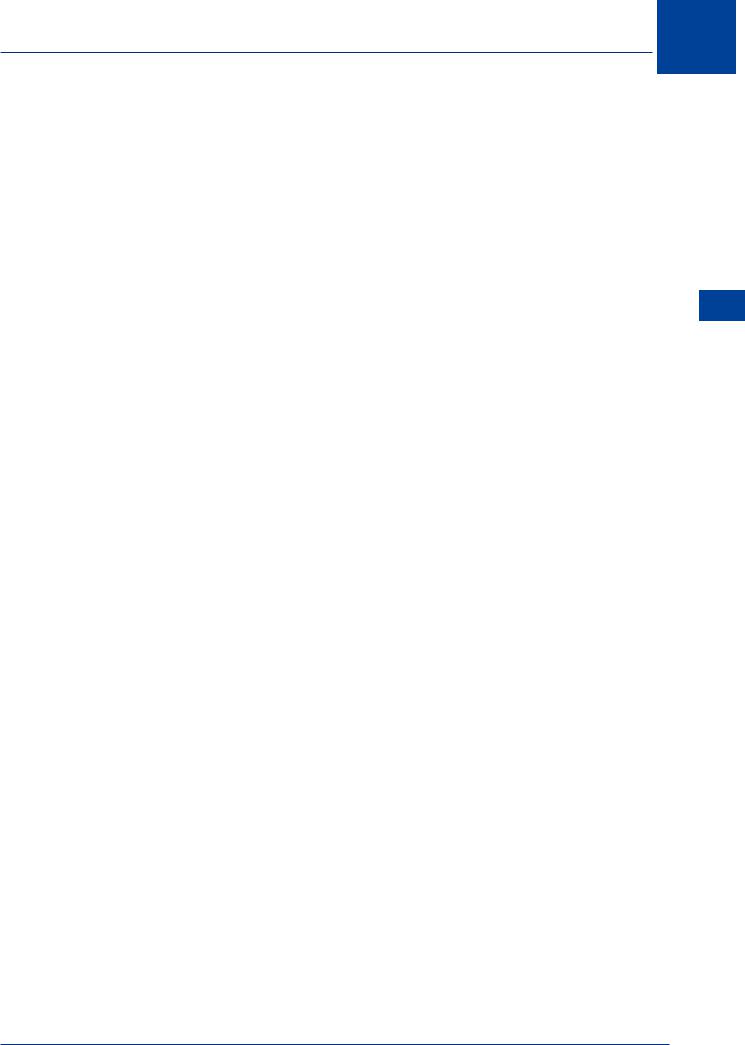
Stalling 7
Spinning
When an aircraft is accidentally or deliberately stalled, the motion of the aircraft may in some cases develop into a spin. The important characteristics of a spin are:
a) |
the aircraft is descending along a steep helical path about a vertical spin axis, |
|
b) |
the angle of attack of both wings is well above the stall angle, |
|
c) |
the aircraft has a high rate of rotation about the vertical spin axis, |
|
d) |
viewed from above, the aircraft executes a circular path about the spin axis, and the |
7 |
|
radius of the helix is usually less than the semi-span of the wing, |
Stalling |
e) |
the aircraft may be in the “erect” or “inverted” position in the spin. |
The spin is one of the most complex of all flight manoeuvres. A spin may be defined as an aggravated stall resulting in autorotation, which means the rotation is stable and will continue due to aerodynamic forces if nothing intervenes. During the spin, the wings remain unequally stalled.
Primary Causes of a Spin
A stall must occur before a spin can take place. A spin occurs when one wing stalls more than the other, Figure 7.32. The wing that is more stalled will drop and the nose of the aircraft will yaw in the direction of the lower wing.
The cause of an accidental spin is exceeding the critical angle of attack while performing a manoeuvre with either too much or not enough rudder input for the amount of aileron being used (crossed-controls). If the correct stall recovery is not initiated promptly, the stall could develop into a spin.
Co-ordinated use of the flight controls is important, especially during flight at low airspeed and high angle of attack. Although most pilots are able to maintain co-ordinated flight during routine manoeuvres, this ability often deteriorates when distractions occur and their attention is divided between important tasks. Distractions that have caused problems include preoccupation with situations inside or outside the flight deck, manoeuvring to avoid other aircraft and manoeuvring to clear obstacles during take-off, climb, approach or landing.
A spin may also develop if forces on the aircraft are unbalanced in other ways, for example, from yaw forces due to an engine failure on a multi-engine aircraft, or if the CG is laterally displaced by an unbalanced fuel load.
183
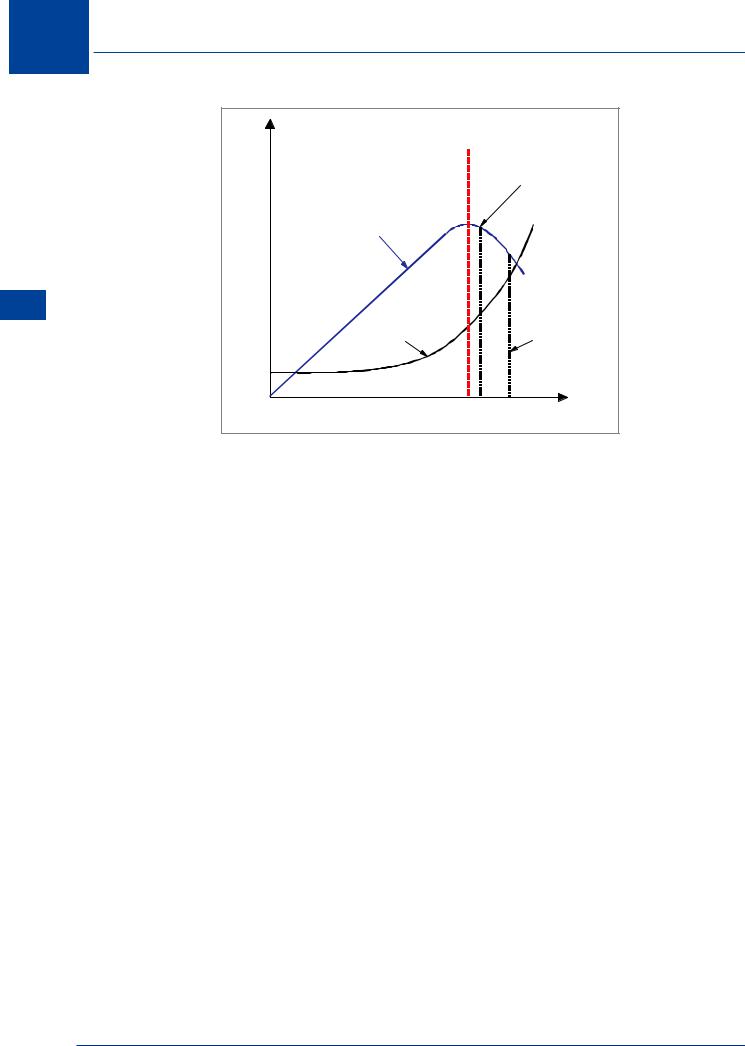
7 |
Stalling |
|
|
STALL |
|
|
|
UPGOING |
|
|
SEMI - SPAN |
|
CL |
|
7 |
|
|
Stalling |
CD |
DOWNGOING |
|
|
SEMI - SPAN |
|
ANGLE OF ATTACK |
|
Figure 7.32
Phases of a Spin
There are three phases of a spin.
1.The incipient spin is the first phase, and exists from the time the aeroplane stalls and rotation starts until the spin is fully developed.
2.A fully developed spin exists from the time the angular rotation rates, airspeed and vertical descent rate are stabilized from one turn to the next.
3.The third phase, spin recovery, begins when the anti-spin forces overcome the pro-spin forces.
If an aircraft is near the critical angle of attack, and more lift is lost from one wing than the other, that wing will drop. Its relative airflow will be inclined upwards, increasing its effective angle of attack. As the aeroplane rolls around its CG, the rising wing has a reduced effective angle of attack and remains less stalled than the other. This situation of unbalanced lift tends to increase as the aeroplane yaws towards the low wing, accelerating the high, outside wing and slowing the inner, lower wing. As with any stall, the nose drops, and as inertia forces begin to take effect, the spin usually stabilizes at a steady rate of rotation and descent.
It is vitally important that recovery from an unintentional spin is begun as soon as possible, since many aeroplanes will not easily recover from a fully developed spin, and others continue for several turns before recovery inputs become effective. Recovery from an incipient spin normally requires less altitude and time than the recovery from a fully developed spin. Every aeroplane spins differently, and an individual aeroplane’s spin characteristics vary depending on configuration, loading and other factors.
184
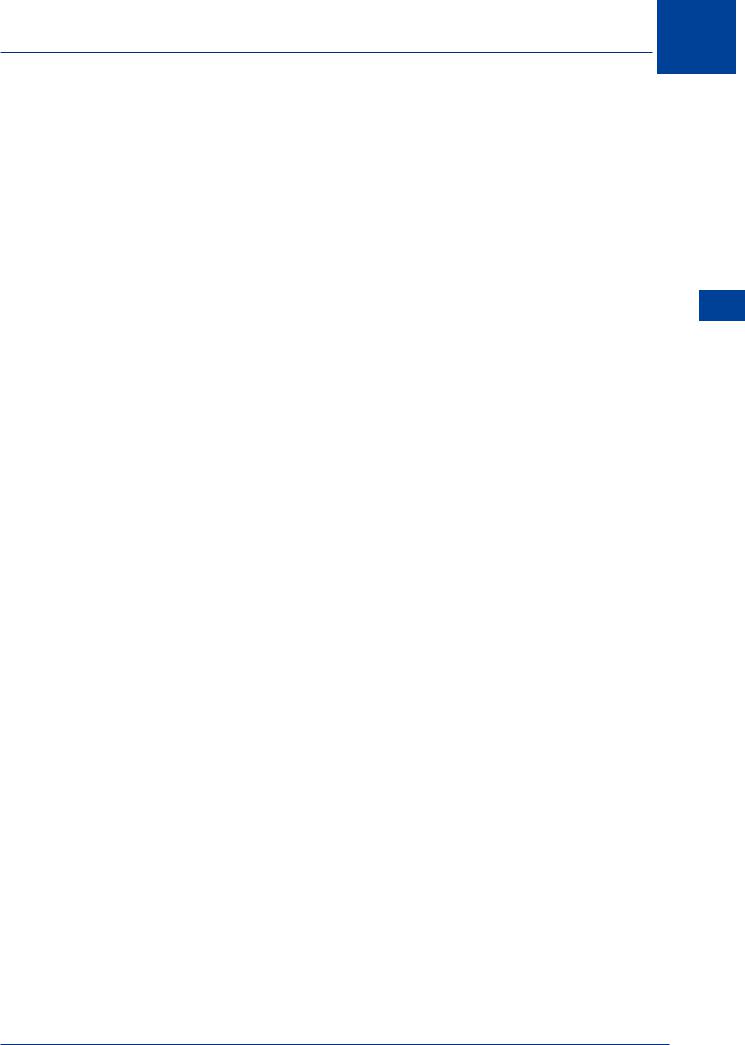
Stalling 7
The Effect of Mass and Balance on Spins
Both the total mass of the aircraft and its distribution influence the spin characteristics of the aeroplane. Higher masses generally mean slower initial spin rates, but as the spin progresses, spin rates may tend to increase. The higher angular momentum extends the time and altitude necessary for recovery from a spin in a heavily loaded aeroplane.
CG location is even more significant, affecting the aeroplane’s resistance to spin as well as all phases of the spin itself.
a) |
CG towards the forward limit makes an aircraft more stable, and control forces will be |
|
|
higher which makes it less likely that large, abrupt control movements will be made. |
7 |
|
When trimmed, the aeroplane will tend to return to level flight if the controls are |
Stalling |
|
released, but the stall speed will be higher. |
|
|
|
|
b) |
CG towards the aft limit decreases longitudinal static stability and reduces pitch control |
|
|
forces, which tends to make the aeroplane easier to stall. Once a spin is entered, the |
|
|
further aft the CG, the flatter the spin attitude. |
|
c) |
If the CG is outside the aft limit, or if power is not reduced promptly, the spin is more |
|
|
likely to go flat. A flat spin is characterized by a near level pitch and roll attitude with |
|
|
the spin axis near the CG. Although the altitude lost in each turn of a flat spin may |
|
|
be less than in a normal spin, the extreme yaw rate (often exceeding 400° per second) |
|
|
results in a high descent rate. The relative airflow in a flat spin is nearly straight up, |
|
|
keeping the wings at high angles of attack. More importantly, the upward flow over |
|
|
the tail may render the elevator and rudder ineffective, making recovery impossible. |
|
Spin Recovery
Recovery from a simple stall is achieved by reducing the angle of attack which restores the airflow over the wing; spin recovery additionally involves stopping the rotation. The extremely complex aerodynamics of a spin may dictate vastly different recovery procedures for different aeroplanes, so no universal spin recovery procedure can exist for all aeroplanes.
The recommended recovery procedure for some aeroplanes is simply to reduce power to idle and release pressure on the controls. At the other extreme, the design of some aircraft is such that recovery from a developed spin requires definite control movements, precisely timed to coincide with certain points in the rotation, for several turns.
185
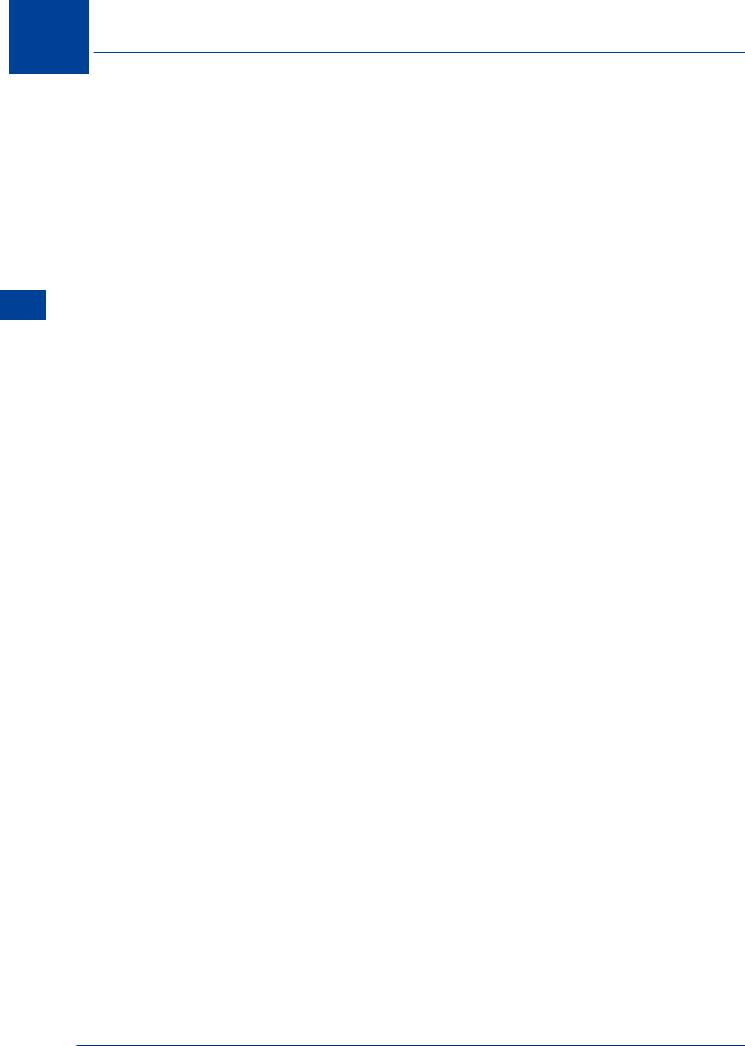
7 Stalling
The following is a general recovery procedure for erect spins. Always refer to the Flight Manual for the particular aircraft being flown and follow the manufacturer’s recommendations.
1.Move the throttle or throttles to idle. This minimizes altitude loss and reduces the possibility of a flat spin developing. It also eliminates possible asymmetric thrust in multi-engine aeroplanes. Engine torque and gyroscopic propeller effect can increase the angle of attack or the rate of rotation in single-engine aeroplanes, aggravating the spin.
2.Neutralize the ailerons. Aileron position is often a contributory factor to flat spins, or to higher rotation rates in normal spins.
7 |
|
3. |
Apply full rudder against the spin. Spin direction is most reliably determined from the |
Stalling |
and may be affected by its location within the flight deck. |
|
turn co-ordinator. Do not use the ball in the slip indicator; its indications are not reliable |
4.Move the elevator control briskly to approximately the neutral position. Some aircraft merely require a relaxation of back pressure, while others require full forward pitch control travel.
The above four items can be accomplished simultaneously.
5.Hold the recommended control positions until rotation stops.
6.As rotation stops, neutralize the rudder. If rudder deflection is maintained after rotation stops, the aircraft may enter a spin in the other direction!
7.Recover from the resulting dive with gradual back pressure on the pitch control.
a)Pulling too hard could trigger a secondary stall, or exceed the limit load factor and damage the aircraft structure.
b)Recovering too slowly from the dive could allow the aeroplane to exceed its airspeed limits, particularly in aerodynamically clean aeroplanes.
Avoiding excessive speed build-up during recovery is another reason for closing the throttles during spin recovery
c)Add power as you resume normal flight, being careful to observe power and RPM limitations.
186