
Cramer C.J. Essentials of Computational Chemistry Theories and Models
.pdf
8.6 GENERAL PERFORMANCE OVERVIEW OF DFT |
281 |
Table 8.1 Mean absolute errors and maximum errors, where available, in atomization energies for different methods over several different test sets (kcal mol−1)
Level of theory |
|
|
|
Test setsa |
|
|
|
|
A |
B |
C |
D |
E |
|
|
|
|
|
|
|
MO theoretical methods |
|
|
|
|
|
|
MNDO |
|
|
|
|
9.3 |
|
|
|
|
|
|
(116.7) |
|
AM1 |
|
|
|
|
7.8 |
|
|
|
|
|
|
(58.2) |
|
PM3 |
|
|
|
|
7.0 |
|
|
|
|
|
|
(32.2) |
|
MNDO/d |
|
|
|
|
7.3 |
|
|
|
|
|
|
(33.9) |
|
HF/6-31G(d) |
85.9 |
80.5 |
150.6b |
|
|
|
|
|
|
(184.3) |
|
|
|
HF/6-31G(d,p) |
|
|
|
119.2 |
|
|
HF/6-311G(2df,p) |
82.0 |
|
146.2b |
|
|
|
HF/6-311+G(3df,2p) |
|
|
74.5 |
144.4b |
148.3c |
211.5c |
|
|
|
(170.0) |
|
(344.1) |
(582.2) |
MP2/6-31G(d) |
22.4 |
16.0 |
38.1b |
|
|
|
|
|
|
(40.3) |
|
|
|
MP2/6-31G(d,p) |
23.7 |
|
22.0 |
|
|
|
MP2/6-311+G(3df,2p) |
|
|
7.3 |
9.7b |
|
|
|
|
|
(25.4) |
|
|
|
MP4/6-31G(2df,p) |
|
|
|
13.5b |
|
|
QCISD/6-31G(d) |
28.8 |
|
51.7b |
|
|
|
MC-QCISD |
|
|
|
1.7b |
|
|
CCSD(T)/6-311G(2df,p) |
11.5 |
|
|
|
|
|
G2(MP2) |
|
|
|
|
1.8 |
|
|
|
|
|
|
(8.8) |
|
CBS-4 |
|
|
|
|
2.7 |
|
|
|
|
|
|
(12.9) |
|
G2 |
1.2 |
1.2 |
|
1.4 |
|
|
|
|
|
(4.9) |
|
(10.6) |
|
CBS-q |
|
|
|
|
2.3 |
|
|
|
|
|
|
(11.4) |
|
CBS-Q |
|
|
1.0 |
1.4b |
1.2 |
|
|
|
|
(3.3) |
|
(8.1) |
|
G3(MP2) |
|
|
|
|
1.2 |
1.2 |
|
|
|
|
|
|
(9.3) |
G3 |
|
|
1.1 |
0.9b |
0.9 |
1.1 |
|
|
|
(4.0) |
|
(4.9) |
(7.1) |
G3S(MP2) |
|
|
|
|
1.2 |
1.3 |
G3S |
|
|
|
0.9b |
1.0 |
1.1 |
MCG3 |
|
|
|
1.0b |
|
|
(continued overleaf )
282 |
8 |
DENSITY FUNCTIONAL THEORY |
|
|
|||
|
|
|
Table 8.1 |
(continued ) |
|
|
|
|
|
|
|
|
|
|
|
Level of theory |
|
|
|
|
Test setsa |
|
|
|
|
|
A |
B |
C |
D |
E |
|
|
|
|
|
|
|
|
W1 |
|
|
|
0.6 |
|
|
|
|
|
|
|
(2.2) |
|
|
|
W2 |
|
|
|
0.5 |
|
|
|
|
|
|
|
(1.9) |
|
|
|
LSDA functionals |
|
|
|
|
|
|
|
SVWN/6-31G(d) |
|
35.7 |
36.4 |
|
83.8 |
|
|
|
|
|
|
(84.0) |
|
|
|
SVWN/6-31G(d,p) |
|
|
|
|
52.2 |
|
|
SVWN/TZ2P |
|
|
|
|
50.1 |
|
|
SVWN/6-311+G(2df,p) |
|
43.5 |
|
|
83.7c |
121.9c |
|
|
|
|
|
|
|
(207.7) |
(347.5) |
GGA and MGGA functionals |
|
|
|
|
|
|
|
BB95/numeric |
|
|
|
8.6 |
|
|
|
BISM/6-311+G(3df,2p) |
|
|
|
(28.6) |
|
|
|
|
|
|
5.9 |
|
|
|
|
|
|
|
|
(21.8) |
|
|
|
BVWN/6-31G(d) |
|
4.4 |
|
|
|
|
|
BKCIS/6-311+G(3df,2p) |
|
|
|
8.2 |
|
14.6 |
|
|
|
|
|
(25.9) |
|
(39.6) |
|
BLYP/6-31G(d) |
|
5.6 |
5.3 |
|
|
|
|
|
|
|
|
(18.8) |
|
|
|
BLYP/6-31G(d,p) |
|
|
|
|
7.0 |
|
|
BLYP/6-31+G(d) |
|
|
|
4.4 |
|
|
|
|
|
|
|
(16.3) |
|
|
|
BLYP/6-311G(2df,p) |
|
9.6 |
|
|
|
|
|
BLYP/6-311+G(3df,2p) |
|
|
|
5.0 |
|
7.3 |
9.3 |
BLYP/6-311++G(3df,3pd) |
|
|
|
(15.8) |
|
(28.4) |
|
|
9.6 |
|
|
7.3c |
9.5c |
||
|
|
|
|
|
|
(28.1) |
(41.0) |
BLYP/pVQZ |
|
|
|
|
7.0d |
|
|
|
|
|
|
|
(27.8) |
|
|
BP86/6-31G(d) |
|
|
|
7.2 |
|
|
|
BP86/6-311+G(3df,2p) |
|
|
|
(24.0) |
|
|
|
|
|
|
10.3 |
|
20.1c |
26.3c |
|
|
|
|
|
(25.4) |
|
(48.7) |
(72.7) |
BPW91/6-31G(d,p) |
|
|
|
|
7.4 |
|
|
BPW91/TZ2P |
|
|
|
|
7.3 |
|
|
BPW91/6-311+G(3df,2p) |
|
|
|
|
|
7.8 |
|
BPW91/6-311++G(3df,3pd) |
|
|
|
|
|
(32.2) |
|
|
6.0 |
|
|
8.0c |
9.0c |
||
EDF1/6-31+G(d) |
|
|
|
|
|
(32.4) |
(28.0) |
|
|
|
3.2 |
|
|
|
|
|
|
|
|
(15.3) |
|
|
|
|
|
|
|
|
|
|
|

8.6 GENERAL PERFORMANCE OVERVIEW OF DFT |
|
283 |
||||||
|
Table 8.1 |
(continued ) |
|
|
|
|
|
|
|
|
|
|
|
|
|
|
|
Level of theory |
|
|
|
Test setsa |
|
|
|
|
|
|
A |
B |
C |
|
D |
E |
|
|
|
|
|
|
|
|
|
|
HCTH/6-311++G(3df,3pd) |
|
|
|
|
|
5.6c |
7.2c |
|
|
|
|
|
|
|
(28.0) |
(28.0) |
|
OLYP/pVQZ |
|
|
|
5.5d |
4.8c |
5.9c |
||
PBE/6-311+G(3df,2p) |
|
|
|
(23.6) |
(27.0) |
(27.0) |
||
|
|
8.2 |
|
|
16.9c |
22.2c |
||
mPBE/6-311++G(3df,3pd) |
|
|
(29.1) |
|
|
(50.5) |
(79.7) |
|
|
|
4.6 |
|
|
6.3 |
|
|
|
PBEKCIS/6-311+G(3df,2p) |
|
|
(18.9) |
|
|
(27.5) |
|
|
|
|
11.9 |
|
|
24.9 |
|
|
|
PKZB/6-311+G(3df,2p) |
|
|
(35.2) |
|
|
(66.5) |
|
|
|
|
3.6 |
|
|
4.8c |
7.0c |
||
PKZBKCIS/6-311+G(3df,2p) |
|
|
(11.0) |
|
|
(39.8) |
(39.8) |
|
|
|
4.1 |
|
|
9.7 |
|
|
|
PWPW91/6-311++G(3df,3pd) |
|
|
(14.4) |
|
|
(38.8) |
|
|
8.6 |
|
|
|
17.7c |
23.6c |
|||
|
|
|
|
|
|
(52.7) |
(81.1) |
|
mPWPW91/6-311++G(3df,3pd) |
6.7 |
|
|
|
15.0c |
|
c |
|
TPSS/6-311++G(3df,3pd) |
|
|
|
|
|
6.0 |
5.8 |
|
VSXC/6-311+G(3df,2p) |
|
|
|
|
|
(22.9) |
(22.9) |
|
|
|
2.5 |
|
|
2.8c |
3.5c |
||
XLYP/6-311+G(3df,2p) |
|
|
(10.0) |
|
|
(11.5) |
(12.0) |
|
|
|
|
|
|
7.6e |
|
|
|
Hybrid functionals |
|
|
|
|
|
|
|
|
BH&HLYP/6-311++G(3df,3pd) |
11.7 |
|
|
|
21.7e |
|
|
|
B0KCIS/6-311+G(3df,2p) |
(23.4) |
|
|
|
|
|
|
|
|
|
3.0 |
|
|
5.3 |
|
|
|
|
|
|
(10.5) |
|
|
(28.6) |
|
|
B1B95/numeric |
|
|
2.0 |
|
|
|
|
|
B1LYP/6-311++G(3df,3pd) |
|
|
(7.5) |
|
|
|
|
|
3.1 |
|
|
|
|
|
|
||
B1PW91/6-311++G(3df,3pd) |
(12.2) |
|
|
|
|
|
|
|
5.4 |
|
|
|
|
|
|
||
|
(14.3) |
|
|
|
|
|
|
|
MPW1K/MG3S |
|
|
|
11.0b |
|
|
|
|
PBE1PBE/6-311+G(3df,2p) |
|
|
3.1 |
4.4b |
4.9c |
6.7c |
||
|
|
|
(10.7) |
|
|
(21.3) |
(35.6) |
|
PW1PW91/6-311+G(3df,2p) |
|
|
|
|
|
e |
|
|
|
|
|
|
b |
5.3e |
|
|
|
mPW1PW91/6-311++G(3df,3pd) |
3.5 |
|
4.2 |
|
3.9 |
|
|
|
TPSSh/6-311++G(3df,3pd) |
(6.7) |
|
|
|
|
|
|
|
|
|
|
|
|
4.2c |
3.9c |
||
|
|
|
|
|
|
(22.0) |
(16.2) |
(continued overleaf )

284 |
8 |
DENSITY FUNCTIONAL THEORY |
|
|
|||
|
|
Table 8.1 |
(continued ) |
|
|
|
|
|
|
|
|
|
|
|
|
Level of theory |
|
|
|
|
Test setsa |
|
|
|
|
|
A |
B |
C |
D |
E |
|
|
|
|
|
|
|
|
B97/numeric |
|
|
|
1.8 |
|
|
|
|
|
|
|
(5.5) |
|
|
|
B97-1/pVQZ |
|
|
|
|
3.2d |
|
|
|
|
|
|
|
(9.8) |
|
|
B3LYP/6-31G(d) |
|
|
|
5.2 |
6.8 |
|
|
B3LYP/6-31+G(d) |
|
|
|
(31.5) |
|
|
|
|
|
|
5.9 |
|
|
|
|
B3LYP/6-311+G(3df,2p) |
|
|
|
(35.9) |
|
|
|
|
|
|
2.4 |
|
3.1 |
4.8 |
|
|
|
|
|
(9.9) |
|
(20.2) |
(21.6) |
B3LYP/aug-cc-pVTZ |
|
|
|
2.6 |
|
|
|
B3LYP/6-311++G(3df,3pd) |
|
|
|
(18.2) |
|
|
|
|
3.3 |
|
4.2b |
3.1c |
4.9c |
||
|
|
|
|
|
|
(20.1) |
(20.8) |
B3LYP/pVQZ |
|
|
|
|
3.4d |
|
|
|
|
|
|
|
(22.2) |
|
|
B3LYP*/TZVPP |
|
|
|
3.0 |
|
|
|
|
|
|
|
(11.1) |
|
|
|
B3P86/6-31G(d) |
|
|
|
5.9 |
|
|
|
B3P86/6-311+G(3df,2p) |
|
|
|
(22.6) |
|
|
|
|
|
|
7.8 |
|
18.2c |
26.1c |
|
|
|
|
|
(22.7) |
|
(48.1) |
(79.2) |
B3PW91/6-31G(d,p) |
|
|
|
|
6.8 |
|
|
B3PW91/TZ2P |
|
|
|
|
6.5 |
|
|
B3PW91/6-311+G(3df,2p) |
|
|
|
|
|
3.5 |
|
B3PW91/6-311++G(3df,3pd) |
|
|
|
|
|
(21.8) |
|
|
4.8 |
|
|
3.4c |
3.9c |
||
|
|
|
|
|
|
(21.6) |
(21.6) |
O3LYP/pVQZ |
|
|
|
|
3.9d |
4.2e |
|
mPW3PW91/6-311++G(3df,3pd) |
|
|
|
(11.8) |
|
|
|
2.7 |
|
|
|
|
|||
X3LYP/6-311+G(3df,2p) |
|
(7.4) |
|
|
|
|
|
|
|
|
|
|
2.8e |
|
a A: G2 subset (32 molecules containing only first-row atoms, see Johnson, Gill, and Pople 1993); B: G2 set (55 molecules including firstand second-row atoms, see Curtiss et al. 1991); C: (108 molecules including firstand second-row atoms, see Scheiner, Baker, and Andzelm 1997); D: G2/97 set (148 molecules including firstand second-row atoms, see Curtiss et al. 1998 and also http://chemistry.anl.gov/compmat/g3theory.htm); E: G3/99 set (223 molecules including firstand second-row atoms, larger organics, and problematic inorganic molecules, see Curtiss et al. 2000).
b Zero-point-exclusive atomization energies for 109 molecules having high overlap with test set C, MG3S basis set for DFT values, see Lynch and Truhlar 2003a.
c Geometries and ZPVE obtained at the B3LYP/6-31G(2df,p) level with scaling of vibrational frequencies by 0.9854; electronic energies use 6-311++G(3df,3pd) basis set; see Staroverov et al. (2003).
d 105 molecules having high overlap with test set C, see Hoe, Cohen, and Handy (2001).
e MP2 geometries and scaled HF thermal contributions; electronic energies use 6-311+G(3df,2p) basis set; see Xu and Goddard (2004a, 2004b).
8.6 GENERAL PERFORMANCE OVERVIEW OF DFT |
285 |
spin-orbit corrections and updated experimental data for the heats of formation of the silicon and beryllium atoms and carbonyl difluoride, while others do not. Readers may refer to the original literature listed in the bibliography for full details.
Some key points may be inferred from Table 8.1.
1.For a given average level of accuracy, hybrid and meta-GGA DFT methods are obviously the most efficient, showing mean unsigned errors almost equal in quality to the much more expensive multilevel correlated methods. However, the maximum absolute errors are larger with the former methods than the latter, indicating a slightly lower generality even in the most current generation of functionals.
2.Hybrid and meta-GGA DFT functionals usually offer some improvement over corresponding pure DFT functionals.
3.Increasing basis-set size does not always improve the accuracy of the DFT models, although it must, of course, ultimately lead to a converged prediction.
4.Of the currently available DFT models, it is clear that GGA models offer a major improvement over the older LSDA model. It further appears that the P86 functional should be avoided. With respect to a more specific ranking of functionals, Boese, Martin and Handy (2003) have carried out a careful evaluation of a large number of functionals
for various energetic quantities (atomization enthalpies, IPs, EAs, geometries, etc.) using polarized triple-ζ basis sets. They conclude that for pure GGA functionals an error ranking (i.e., lower is better) is HCTH < OLYP < BPW91 < BLYP < mPWPW91 < BP86 < PWPW91 < PBE. They further conclude that for hybrid and meta-GGA functionals, which are overall better than pure functionals, an analogous error ranking is hybrid τ -HCTH < B97-1 < τ -HCTH < VSXC B97-2 B3LYP B98 < PBE1PBE << MPW1K < PKZB (note that these comparisons do not take into account predicted reaction activation enthalpies). Prior work by Proynov, Chermette, and Salahub (2000) suggests that Bmτ 1 and BLap3 have qualities intermediate between B3LYP and PBE1PBE in the latter rank ordering.
While fewer data are available, the utility of DFT in computing the bond strengths between transition metals and hydrides, methyl groups, and methylene groups has also been demonstrated (Table 8.2). Because of the non-dynamical correlation problem associated with the partially filled metal d orbitals, such binding energies are usually very poorly predicted by MO theory methods, until quite high levels are used to account for electron correlation.
In the area of reaction energetics, Baker, Muir, and Andzelm have compared six levels of theory for the enthalpies of forward activation and reaction for 12 organic reactions: the unimolecular rearrangements vinyl alcohol → acetaldehyde, cyclobutene → s-trans butadiene, s-cis butadiene → s-trans butadiene, and cyclopropyl radical → allyl radical; the unimolecular decompositions tetrazine → 2HCN + N2 and trifluoromethanol → carbonyl difluoride + HF; the bimolecular condensation reactions butadiene + ethylene → cyclohexene (the Diels–Alder reaction), methyl radical + ethylene → propyl radical, and methyl radical + formaldehyde → ethoxyl radical; and the bimolecular exchange reactions FO + H2 → FOH + H, HO + H2 → H2O + H, and H + acetylene → H2 + HC2. Their results are summarized in Table 8.3 (Reaction Set 1). One feature noted by these authors is
286 |
|
|
8 DENSITY FUNCTIONAL THEORY |
|
||
|
Table 8.2 Mean absolute errors in metal – ligand binding |
|||||
|
energies for different methods (kcal mol−1)a |
|
||||
|
Level of theoryb |
|
Ligand |
|
||
|
|
|
|
H |
CH3 |
CH2 |
|
|
|
|
|
||
|
MO theoretical methods |
|
|
|
||
|
MCPF |
|
6 |
9 |
20 |
|
|
QCISD(T) |
|
6 |
|
||
|
PCI-80 |
|
2 |
2 |
4 |
|
|
Density functional methods |
|
|
|
||
|
SVWN |
|
12 |
|
|
|
|
BP86 |
|
|
8 |
|
|
|
BH&HLYP |
|
|
16 |
||
|
BH&HLYP/ECP |
|
5 |
9 |
||
|
B3LYP |
|
5 |
6 |
4 |
|
|
B3LYP/ECP |
|
9 |
7 |
||
|
|
|||||
|
a Complexes MX+ where M = Sc, Ti, V, Cr, Mn, Fe, Co, Ni, Cu, |
|||||
|
b |
= |
ligand. |
|
|
|
|
and X |
|
|
|
|
Basis sets are for the most part double-ζ polarized in quality. Use of metal effective core potentials is indicated by ECP.
that the pure DFT functional BLYP badly underestimated the activation enthalpies for the H-atom transfer reactions. This behavior has since been noted by many other authors, and is part of the motivation for the MPW1K model discussed in Section 8.4.3.
In a different analysis of hydrogen-atom transfer reaction barriers, Lynch and Truhlar (2003b) considered the forward and reverse barriers for 22 such reactions, and then demonstrated that the performance of various models on only six data (the forward and reverse barrier heights for HO + CH4 → H2O + CH3, H + OH → O + H2, and H + H2S → H2 + HS) was highly predictive of their accuracy for all 44 barrier heights. In this and subsequent work by Zhao et al. (2004), they assayed the accuracy of a substantial number of different models for this test set and found that modern functionals, and particularly those including a dependence on the kinetic energy density, gave the best results (Table 8.3, Reaction Set 2). However, all of the geometries used in this analysis were obtained at the QCISD/MG3 level (partly in recognition of the multideterminantal character of many H-atom transition-state structures). So, the reported errors might be expected to change somewhat were geometries to be located at the DFT levels of theory. In this regard, it is noteworthy that Kang and Musgrave (2001) examined 29 different barrier heights for H-atom transfer using geometries optimized at the same level as used to compute energies, and reported mean unsigned errors of 0.9, 3.3, 3.2, and 2.9 kcal mol−1, respectively, for KMLYP, B3LYP, BH&HLYP, and G2.
With respect to chemical reactions not involving H-atom transfer, Guner et al. (2003, 2004) examined a set of 11 pericyclic organic reactions having experimental data available for nine enthalpies of activation and six enthalpies of forward reaction. They found the B3LYP and MPW1K functionals to be about as accurate for the activation enthalpies as the more expensive CBS-QB3 and CASPT2 levels, with other functionals doing somewhat less well (Table 8.3, Reaction Set 3). Interestingly, they found the performance of the B3LYP

8.6 GENERAL PERFORMANCE OVERVIEW OF DFT |
287 |
Table 8.3 Mean and maximum absolute errors (kcal mol−1) in enthalpies of activation and forward reaction for different methods
Level of theory |
|
Activation |
|
|
Reaction |
|
Mean |
Maximum |
Mean |
Maximum |
|
|
|
|
|
|
|
Reaction set 1a,b |
|
|
|
|
|
MNDO |
23.4 |
51.8 |
10.9 |
57.7 |
|
AM1 |
9.3 |
34.2 |
7.5 |
22.1 |
|
HF/6-31G(d)c |
13.6 |
30.6 |
10.5 |
24.8 |
|
MP2 |
9.9 |
28.8 |
6.3 |
26.0 |
|
BLYP |
5.9 |
21.9 |
5.9 |
13.0 |
|
BLYP/6-311G(2df,2pd) |
|
|
5.8 |
16.0 |
|
B3LYP |
|
|
5.0 |
9.4 |
|
B3LYP/6-311G(2df,2pd) |
|
|
3.7 |
8.5 |
|
B3PW91 |
3.7 |
12.9 |
6.8 |
17.7 |
|
OLYP |
|
|
4.6 |
12.7 |
|
OLYP/6-311G(2df,2pd) |
|
|
3.6 |
10.6 |
|
O3LYP |
|
|
4.6 |
11.6 |
|
O3LYP/6-311G(2df,2pd) |
|
|
2.8 |
7.1 |
|
Reaction set 2d |
|
|
|
|
|
HF |
12.4 |
|
149.5 |
|
|
MP2 |
5.5 |
|
24.4 |
|
|
QCISD |
3.9 |
|
38.6 |
|
|
QCISD(T) |
3.1 |
|
32.3 |
|
|
CBS-Q |
0.8 |
|
1.3 |
|
|
MC-QCISD |
0.9 |
|
1.1 |
|
|
MCG3 |
0.8 |
|
0.8 |
|
|
BB95 |
8.3 |
|
8.2 |
|
|
BLYP |
8.3 |
|
6.8 |
|
|
BP86 |
9.4 |
|
13.0 |
|
|
G96LYP |
6.9 |
|
11.1 |
|
|
HCTH |
5.4 |
|
5.3 |
|
|
mPWPW91 |
8.6 |
|
7.2 |
|
|
OLYP |
6.0 |
|
3.4 |
|
|
PBE |
9.5 |
|
12.1 |
|
|
PWPW91 |
9.8 |
|
12.1 |
|
|
VSXC |
5.1 |
|
3.4 |
|
|
B1B95 |
3.7 |
|
3.4 |
|
|
B97-1 |
4.2 |
|
5.8 |
|
|
B97-2 |
3.1 |
|
3.9 |
|
|
B98 |
4.1 |
|
6.3 |
|
|
KMLYP |
2.9 |
|
1.0 |
|
|
mPW1PW91 |
3.9 |
|
8.0 |
|
|
MPW1K |
1.4 |
|
14.9 |
|
|
PBE1PBE |
4.6 |
|
7.1 |
|
|
B3LYP |
5.0 |
|
7.2 |
|
(continued overleaf )

288 |
8 DENSITY FUNCTIONAL THEORY |
|
|
|
|||
|
|
Table 8.3 |
(continued ) |
|
|
|
|
|
|
|
|
|
|
|
|
Level of theory |
|
Activation |
|
|
Reaction |
||
|
|
|
|
|
|
|
|
|
|
Mean |
Maximum |
Mean |
Maximum |
||
|
|
|
|
|
|
||
B3PW91 |
4.4 |
|
|
5.8 |
|
||
O3LYP |
4.7 |
|
|
5.8 |
|
||
Reaction set 3b,e |
|
|
|
|
|
|
|
HF |
18.7 |
26.7 |
|
3.8 |
6.5 |
||
CASSCF |
16.0 |
34.6 |
|
14.7 |
20.6 |
||
MP2 |
4.6 |
7.6 |
|
6.0 |
9.6 |
||
CASPT2//CASSCF |
2.4 |
5.7 |
|
1.6 |
4.5 |
||
CBS-QB3 |
1.9 |
4.3 |
|
1.6 |
2.5 |
||
BPW91 |
3.7 |
6.9 |
|
3.4 |
7.4 |
||
KMLYP |
3.2 |
10.3 |
|
12.7 |
19.8 |
||
OLYP |
3.4 |
9.0 |
|
6.2 |
12.9 |
||
OLYP/6-311+G(2d,p) |
4.4 |
13.0 |
|
9.8 |
20.5 |
||
MPW1K/6-31+G(d,p) |
2.2 |
6.9 |
|
6.2 |
10.0 |
||
B3LYP |
1.7 |
6.0 |
|
4.1 |
8.6 |
||
B3LYP/6-31+G(d,p) |
2.4 |
8.1 |
|
7.0 |
13.6 |
||
B3LYP/6-311+G(2d,p) |
2.9 |
10.1 |
|
8.2 |
15.9 |
||
O3LYP//OLYP |
3.0 |
9.0 |
|
3.9 |
8.3 |
a See Baker, Muir, and Andzelm (1995).
b 6-31G(d) basis set unless otherwise indicated.
c Using five spherical d functions instead of the usual six Cartesian functions implied by this basis set name.
d See Lynch and Truhlar (2003a) and Zhao et al. (2004); 6-31+G(d,p) basis set; the Reaction column refers to the atomization enthalpies for six molecules chosen to be representative of a larger set in a fashion analogous to the H-atom transfer reactions, namely, SiO, S2, silane, propyne, glyoxal, and cyclobutane.
e See Guner et al. (2003, 2004).
functional to become systematically worse with increasing basis-set size, so some cancellation of errors appears to be responsible for the excellent performance of this functional when used with the 6-31G(d) basis set. This analysis is supported as well by the relatively poor performance of B3LYP for the forward reaction enthalpies. Indeed, although the best MO methods continue to perform well for this latter test, the best DFT functional was found to be BPW91, which was the worst for the activation enthalpies.
St-Amant et al. (1995) have analyzed the utility of HF, MP2, LSDA, and BP86, using basis sets of DZP to TZP quality, for the prediction of 35 conformational energy differences in small to medium-sized organic molecules (e.g., axial–equatorial disposition of substituents on cyclohexanes, E vs. Z amide rotamers, s-cis vs. s-trans rotamers of carboxylic acids and conjugated systems, etc.) The mean unsigned errors over the data for these four methods are 0.5, 0.4, 0.6, and 0.3 kcal mol−1, respectively; the average conformational energy difference is 1.6 kcal mol−1.
A substantial body of data exists evaluating the utility of DFT (and other methods) for computing ionization potentials and electron affinities following a SCF approach. These data are summarized over four different test sets in Table 8.4. The conclusions one may
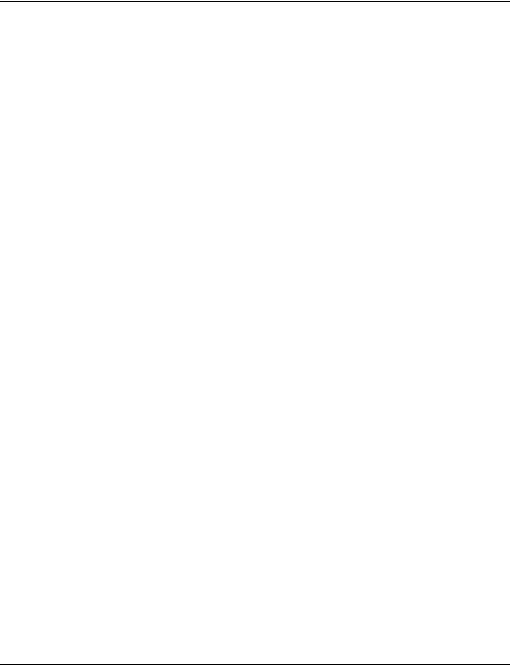
8.6 GENERAL PERFORMANCE OVERVIEW OF DFT |
289 |
Table 8.4 Mean absolute errors and maximum errors, where available, in IPs and EAs for different methods over several different test sets (eV)
Level of theory |
|
IP test sets |
|
EA test sets |
|
|
G2a |
G2/97b |
|
G2 |
G2/97 |
|
|
|
|
|
|
MO theoretical methods |
|
|
|
|
|
HF |
|
1.03c |
|
|
1.10c |
|
|
(2.60) |
|
|
(2.21) |
G2(MP2) |
0.1 |
|
0.1 |
|
|
G2 |
0.06 |
0.06 |
0.06 |
0.06 |
|
|
(0.19) |
(0.19) |
(0.14) |
(0.17) |
|
CBS-QB3 |
0.05 |
|
0.05 |
|
|
|
(0.12) |
|
(0.12) |
|
|
G3 |
0.04 |
0.04 |
0.05 |
0.04 |
|
|
(0.18) |
(0.18) |
(0.18) |
(0.18) |
|
W1 |
0.01 |
0.02 |
0.02 |
0.02 |
|
|
(0.06) |
(0.13) |
(0.05) |
(0.08) |
|
W2 |
0.01 |
|
0.01 |
|
|
|
(0.05) |
|
(0.04) |
|
|
LSDA functionals |
|
|
|
|
|
SVWN/6-311+G(2df,p) |
0.7 |
0.6 |
0.7 |
0.7 |
|
|
(1.2) |
(1.7) |
(1.2) |
(1.3) |
|
SVWN/aug-cc-pVTZ |
0.7 |
|
0.8 |
|
|
SVWN5/6-311+G(2df,p) |
0.2 |
0.23c |
0.3 |
0.24c |
|
|
(0.6) |
(1.18) |
(0.7) |
(0.88) |
|
GGA and MGGA functionals |
|
|
|
|
|
BB95/numeric |
0.2 |
|
|
|
|
BLYP/6-311+G(3df,2p) |
(0.5) |
|
|
|
|
0.19 |
0.29c |
0.11 |
0.12c |
||
|
(0.6) |
(1.06) |
(0.4) |
(0.70) |
|
BLYP/aug-cc-pVTZ |
0.2 |
|
0.1 |
|
|
BP86/6-311+G(3df,2p) |
0.18 |
0.22c |
0.21 |
0.19c |
|
|
|
(1.21) |
|
|
(0.89) |
BP86/aug-cc-pVTZ |
0.2 |
|
0.2 |
|
|
BPW91/6-311+G(3df,2p) |
0.16 |
0.24c |
0.09 |
0.12c |
|
HCTH/6-311++G(3df,3pd) |
|
(1.14) |
|
|
(0.78) |
|
0.23c |
|
|
|
|
OLYP/6-311++G(3df,3pd) |
|
(1.34) |
|
|
|
|
0.29c |
|
|
0.15c |
|
PBE/6-311+G(3df,2p) |
|
(1.11) |
|
|
(0.60) |
0.16 |
0.24c |
0.12 |
0.12c |
||
PKZB/6-311++G(3df,3pd) |
(0.5) |
(1.11) |
(0.3) |
(0.78) |
|
|
0.31c |
|
|
0.15c |
|
PWPW91/6-311++G(3df,3pd) |
|
(1.31) |
|
|
(0.57) |
0.16 |
0.22c |
0.14 |
|
||
|
|
(1.19) |
|
|
|
(continued overleaf )
