
Baumgarten
.pdf
6.4 Homogeneous Charge Compression Ignition (HCCI) 275
Fig. 6.41. Effect of inlet air temperature on HCCI combustion [94]
Air Excess Ratio
As already described together with the effects of EGR, progressively richer mixtures translate into advancing ignition and faster energy release. At a certain limit, depending on the fuel and further boundary conditions, combustion stability degrades, knock appears and the NOx emissions increase [23, 44, 31]. On the other hand, leaner mixtures deliver retarded ignition and slower energy release. Extremely lean mixtures result in incomplete combustion at low temperatures leading to increased emissions of CO and HC. Supercharging can increase the IMEP of the engine under HCCI operation if combined with heavy EGR. However, due to the low exhaust gas temperatures, the energy provided by the exhaust gas turbine of the turbocharger is often not sufficient to realize high boost pressures [73].
Engine Speed
In conventional engines, the increasing engine speed results in increased turbulence and burn rates, and thus no measurable effect of speed can be reported. With HCCI systems however, the mixture homogeneity is already completed prior to combustion. This is the reason why reaction rates remain relatively unchanged with varying engine speed and thus the time available for reactions is reduced with increasing engine speed. High engine speed can therefore result in misfire and in reduced power output and efficiency [67, 91].
Fuel Composition
Regarding the HCCI combustion process, fuels can be divided into two main groups, the diesel-like fuels and the gasoline-like ones. Diesel-like fuels (increased


6.4 Homogeneous Charge Compression Ignition (HCCI) 277
Fig. 6.43. Comparison of oxidation process for different fuels (data from [92])
straight-chain saturated hydrocarbons (n-pentane, n-hexane, n-heptane) with different chain lengths. The engine used in this study was a 2.0 liter four-valve naturally aspirated single-cylinder engine with a compression ratio of 16.5:1. The complete homogenization of the mixture was performed in an external vaporizer, and intake temperature (100°C) as well as equivalence ratio and O2-concentration were kept constant for all experiments. All fuels show the characteristic two-stage combustion with LTO and HTO, Fig. 6.42a. The ignition and combustion behavior depends on the fuel molecular size: the longer the carbon chain and thus the higher the ignitability (longer molecules break up more easily into radicals), the earlier the start of LTO and the more energy is released. For this reason, the mean temperature in the combustion chamber earlier reaches the value of approximately 900 K, which is needed to initiate the HTO.
A more detailed description of these effects is given in Figs. 6.42b and 6.42c. Figure 6.42b shows the fuel concentrations and mean fuel temperatures versus crank angle, and Fig. 6.42c presents the consumption of oxygen. The fuel concentrations start to decrease at about 700 K. The longer the carbon chain, the earlier the fuel breaks up into radicals and the faster the reactions. This results in an earlier and faster decrease of fuel concentration combined with an earlier and faster consumption of oxygen, and a higher amount of heat is released as proven by the temperature curves. The investigations show that for n-paraffin fuels, the fuel having a longer straight chain is more likely to be oxidized in the low temperature range up to 900 K. The curves of fuel and oxygen concentration change their respective gradients at a temperature of 900 K, which is the start of HTO. From this point on, the gradients are the same for all fuels, and the effect of molecular size is negligible.
The investigations suggest that it is possible to control the oxidation rate in the low temperature range by modifying the fuel. For this reason, Tsurushima et al. [92] have also performed investigations with a mixture of n-hexane and ethylene. The resulting heat release again shows the well-known two-stage behavior, Fig. 6.43, but the behavior of both fuel components during the first stage (LTO) is dif-
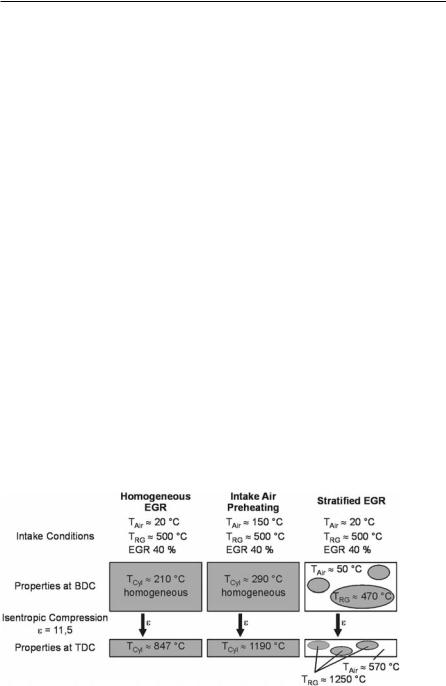

6.4 Homogeneous Charge Compression Ignition (HCCI) 279
Partly homogenized mixtures on the other hand have a higher probability to ignite earlier if hot EGR zones adjoin to fuel-rich reactive zones. In this case, the ignition occurs at overall lower but sufficiently high local temperatures without heating of the intake air. Ignition occurs first in a relatively small area, but because the whole charge is near the ignition limit, there is no conventional flame combustion. The energy release of the first local ignition initiates a multitude of further ignitions in the whole combustion space resulting in a homogeneous combustion, the energy release rates of which can be controlled by the degree of homogenization [94].
6.4.6 Transient Behavior – Control Strategies
As soon as steady-state conditions are achieved, the HCCI combustion is remarkable stable, but small challenges in the boundary conditions have a significant negative impact on the engine behavior. Unfortunately, in real engine operation the parameters described in the previous section, which are used to control the HCCI combustion process, are interacting strongly. Conventional single input – single output control strategies cannot be applied for a sufficient control under transient conditions any more [23]. Besides conventional and relatively slow mass flow, air excess ratio, and temperature sensors, a real-time combustion signal is needed in order to control the combustion from cycle to cycle and to allow a transient variation of speed and load in the HCCI operation region. One of the most challenging tasks is the mode transition between HCCI and conventional combustion. In this case, the change of the relevant thermodynamic values is unsteady from one cycle to the other, and a model-based combustion control with a precise prediction of charge composition and thermodynamic conditions is required. In the case of a dual-mode CAI engine for example, the transition from SI to HCCI mode must be realized if load is reduced. Due to the typically high exhaust gas temperatures there will be a very advanced combustion with an unfavorable maximum pressure rise in the first HCCI cycle. The sudden change from one mode to the other has to be smoothened by a model-based transition functionality.
6.4.7 Future HCCI Engine Applications
HCCI engines have demonstrated their potential to realize very low emissions of NOx and particulate matter (PM), as well as high thermal efficiency. However, in order to realize these advantages in modern engines, numerous problems still have to be solved. Although full-time HCCI engines have the biggest theoretical potential to exploit the benefits of HCCI-combustion, it is still in question if this combustion concept will ever function at full load. Today, HCCI applications are limited to part load, but it is expected that the further development of special tailored HCCI-fuels and combustion phasing control might help to expand the area of HCCI-combustion in the engine map. Some authors even predict that future development will result in a so-called Combined Combustion System (CCS) [5, 82],


References 281
[6]Allen J, Law D (2001) Advanced Combustion Using a LOTUS Active Valve Train, Internal Exhaust Gas Recirculation Promoted Auto-Ingition. In: Duret P (ed) A New Generation of Engine Combustion Processes for the Future?, Paris, pp 85–100
[7]Aoyama T, Hattori Y, Mizuta J, Sato Y (1996) An Experimental Study on PremixedCharge Compression Ignition Gasoline Engines. SAE paper 960081
[8]Böhm H Hesse D, Jander H, Lüers B, Pietscher J, Wagner HG, Weiss M (1988) The Influence of Pressure and Temperature on Soot Formation in Premixed Flames. 22nd Symposium (International) on Combustion, The Combustion Institute, pp 403–411
[9]Bonse B, Dittus B, Giersch J, Kerst A, Kügler T, Schuhmacher H, Wintrich T (2003) Diesel Injection Nozzle – Innovations with Opportunities to Reduce Emissions, Fuel Consumption and Noise. 5. Int Stuttgarter Motorensymposium, Stuttgart, pp. 1933
[10]Chevalier C, Louessard P, Müller UC, Warnatz J (1990) A Detailed LowTemperature Reaction Mechanism of n-Heptane Auto-Ignition. Proceedings of the 2nd Symposium on Diagnostics and Modeling of Combustion in Reciprocating Engines, Comodia 90, pp 93–97
[11]Chevalier C, Warnatz J, Melenk H (1990) Automatic Generation of Reaction Mechanisms for the Description of the Oxidation in Higher Hydrocarbons. Berichte der Bunsen-Gesellschaft für Physikalische Chemie vol 94, pp 1362–1367
[12]Chmela F, Jager P, Herzog P, Wirbeleit F (1999) Emissionsverbesserung an Dieselmotoren mit Direkteinspritzung mittels Einspritzverlaufsformung. Motortechnische Zeitschrift (MTZ) 60, pp. 552–558. English Version: Reducing Exhaust Emissions of Direct Injection Diesel Engines via Injection Rate Shaping. MTZ worldwide, 60 (9/1999), pp. 5–8
[13]Curran HJ, Gaffuri P, Pitz WJ, Westbrook CK (1998) A Comprehensive Modeling Study of n-Heptane Oxidation. Combust. Flame 114, pp 149–177
[14]Dohle U, Dürnholz M, Kampmann S, Hammer J, Hinrichsen C (2004) 4th Generation Diesel Common-Rail Injection System for Future Emission Legislation. FISITA World Automotive Congress, Barcelona, paper F2004V271
[15]Dohle, U (2003) Innovative Injection Technology for Future Diesel Passenger Cars. 12th Aachener Motorenkolloquium, Aachen, pp. 107–123
[16]Drake MJ, Ratcliffe JW, Blint RJ, Carter CD, Laurendeau NM (1990) Measurements and Modelling of Flamefront NO Formation and Superequilibrium Radical Concentrations in Laminar High-Pressure Premixed Flames. 23th Symposium (International) on Combustion, The Combustion Institute, Pittsburgh, pp 387–395
[17]Duret P, Gatellier B, Miche M, Montreiro L, Zima P, Marotaux D, Blundell D, Ganser M, Zhao H, Perozzi M, Araneo L (2003) Innovative Diesel HCCI Combustion Process for Passenger Cars: the European SPACE LIGHT Project. EAEC Congress, paper C108
[18]Ehlers G, Pfalzgraf B, Wurms R (2003) Homogeneous Split – a Highly Efficient Strategy to Reduce the Exhaust Emissions of FSI Engines. 9th Symposium The Working Process of the Internal Combustion Engine, Institute for Internal Combustion Engines and Thermodynamics, Graz University of Technology, Austria
[19]Eichlseder H, Baumann E, Müller P, Neugebauer S (2000) Chancen und Risiken von Ottomotoren mit Direkteinspritzung. Motortechnische Zeitschrift (MTZ), 61 (3/2000), pp 144–152. English version: Potential and Risks of Gasoline Direct Injection Engines for Passenger Car Drivelines. MTZ worldwide, 61 (3/2000), pp 2–5
[20]Epping K, Aceves S Bechtold R, Dec J (2002) The Potential of HCCI Combustion for High Efficiency and Low Emissions. SAE paper 2002-01-1923


References 283
[38]Ishibashi Y, Asai M (1996) Improving the Exhaust Emission of Two-Stroke Engines by Applying the Activated Radical Combustion. SAE paper 960742
[39]Iwabuchi Y, Kawai K, Shoji T, Takeda Y (1999) Trial of New Concept Diesel Combustion System – Premixed Compression-Ignited Combustion. SAE paper 1000-01- 0185
[40]Jorach RW, Doppler H, Altmann O (2000) Schweröl-Common-Rail- Einspritzsysteme für Großmotoren. Motortechnische Zeitschrift (MTZ) 61, pp. 854-861. English Version: Heavy Fuel Common Rail Injection Systems for Large Engines. MTZ worldwide, 61 (12/2000) 4, pp. 10–13
[41]Kammerdiener T, Bürgler L (2000) Ein Common-Rail Konzept mit druckmodulierter Einspritzung. Motortechnische Zeitschrift (MTZ) 61, pp 230–238. English Version: A Common Rail Concept with Pressure-Modulated Fuel Injection. MTZ worldwide,
61(4/2000), pp. 7–11
[42]Kelly-Zion PL, Dec JE (2000) A Computational Study on the Effect of Fuel-Type on Ignition Time in HCCI Engines. Proc. Combust. Inst. 28, paper no. 4E11
[43]Kimura S, Aoki O, Kitahara Y, Aijoshizawa E (2001) Ultra-Clean Combustion Technology Combining a Low-Temperature and Premixed Combustion Concept for Meeting Future Emission Standards. SAE paper 2001-01-0200
[44]Kowalewicz A (1984) Combustion Systems of High Speed Piston I.C. Engines. Wydawnictwa Komunikacji, Warsaw
[45]Kraft M, Maigaard P, Mauss F, Christensen M, Johansson B (2000) Investigation of Combustion Emissions in a HCCI Engine – Measurements and a New Computational Model. Proc. Combust. Inst. 28, paper no. 4E12
[46]Kufferath A, Samenfink W, Gerhardt J (2003) Die neue Emissionsstrategie der Ben- zin-Direkteinspritzung. Motortechnische Zeitschrift (MTZ), 64 (11/2003), pp 916–
923.English version: The new Emissions Strategy of Gasoline Direct Injection. MTZ worldwide 64 (11/2003), pp 6–9
[47]Lavoie GA, Heywood JB, Keck JC (1970) Experimental and Theoretical Study of Nitric Oxide Formation in Internal Combustion Engines. Combustion Science and Technology, vol 1, pp 313–326
[48]Law D, Allen J (2002) On the Mechanism of Controlled Auto Ignition. SAE paper 2002-01-0421
[49]Law D, Kemp D, Allen J, Kirkpatric G, Copland T (2000) Controlled Combustion in an IC-Engine with a Fully Variable Valve Train. SAE paper 2000-01-0251
[50]Lettmann H, Seebode J, Merker GP (2004) Numerical and Experimental Evaluation of DI-Diesel Soot and Nitrogen Oxide Emissions in Respect of Different Injection Strategies. FISITA World Automotive Congress, Barcelona, paper F2004V240
[51]Li H, Miller DL, Cernansky NP (1996) Development of Reduced Kinetic Model for Prediction of Preignition Reactivity and Autoignition of Primary Reference Fuels. SAE paper 960498
[52]Mahr, B (2002) Future and Potential Diesel Injection Systems. THIESEL 2002 Conference on Thermoand Fluid-Dynamic Processes in Diesel Engines
[53]Malte PC, Pratt DT (1974) Measurement of Atomic Oxygen and Nitrogen Oxides in Jet-Stirred Combustion. 15th Symposium (International) on Combustion, The Combustion Institute, Pittsburgh, pp 1067–1070
[54]Meyer S, Krause A, Krome D, Merker GP (2002) Ein flexibles Piezo-Common-Rail System mit direktgesteuerter Düsennadel (in German). Motortechnische Zeitschrift (MTZ) 2/2002 Jahrgang 63, pp 86–93, also available in English (MTZ Worldwide)
