
Ellinger Y., Defranceschi M. (eds.) Strategies and applications in quantum chemistry (Kluwer, 200
.pdf436 |
A. RAHMOUNI AND C. BARBIER |
In order to compare the electronic properties of the (001), (010) and (100) faces three
clusters have been selected each one modelling one specific face of the |
crystal, these |
|
clusters contain the same number of oxygen and molybdenum atoms : |
and |
for |
the (001), (010) and (100) faces respectively. |
|
|
The total energy values (Table 6) show that the (010) face is more stable than the (001) and (100) faces, in agreement with the results of Firment[21], also our calculations show that the (001) face is more stable than the (100) one.
FROM CLUSTER TO INFINITE SOLID |
437 |
Since the type of oxygen vacancy depends on the (001) or (100) faces, the unsaturated molybdenum charge is highest in the negative charge of unsaturated oxygen is higher than in the coordinatively saturated atoms. Analysis of the Mo-O overlap population shows that the bond is stronger on the (100) face whereas the other Mo-O bonds are stronger on the (001) face.
438 |
A. RAHMOUNI AND C. BARBIER |
4. Conclusion
The similarity of the results obtained for finite clusters and the infinite slab allows to conclude in favour of the validity of the cluster model of adequate size (6 or 8 molybdenum atoms). In addition to the chemisorption of organic molecules on solid surfaces which is generally considered as a localized phenomenon, the interaction between molybdenum oxide and an adsorbate can also be represented by a local complex formed by a finite cluster and the adsorbed molecule. Indeed, the study of the evolution of the electronic properties as a function of the cluster size shows that, for a cluster containing 6 or 8 molybdenum atoms, most of the electronic properties converge towards limit values. This convergence is sensitive to the direction of the cluster growth. On the other hand, the electronic properties of the (001), (010) and (100) faces are not identical, the type of surface atoms being different ; these results allow to predict that the characteristics of the chemisorption step will depend on the particular face on which it takes place.
Acknowledgement
The authors gratefully acknowledge technical assistance of the "Centre d'Informatique Scientifique et Médical" of the University Claude Bernard Lyon I.
References
1.R. Hoffmann, J. Chem. Phys. 39, 1397 (1963).
2.A. B. Anderson, Y. Kim, D. W. Ewing, R. K. Grasselli and M. Tenhover, Surf. Sci., 134, 237 (1983).
3.J. B. Moffat, J. Mol. Catal. 260, 385 (1984).
4.D. Masure, P. Chaquin, C. Louis, M. Che and M. Fournier, J. Catal. 119, 415 (1989).
5.M. Fournier, C. Louis, M. Che, P. Chaquin and D. Masure, J. Catal. 119, 400
(1989).
6.J. Sylvestre, J. Am. Chem. Soc. 109, 594 (1987).
7.M.-H. Whangbo and L. F. Schneemeyer, Inorg. Chem. 25, 2424 (1986).
8.M.-H. Whangbo and E. Canadell, J. Am. Chem. Soc. 110, 358 (1988).
9.M.-H. Whangbo, E. Canadell and C. Schlenker, J. Am. Chem. Soc. 109, 6308 (1987).
10.E. Canadell and M.-H. Whangbo, Inorg. Chem. 27, 228 (1988).
11. |
E. Canadell, M.-H. Whangbo, C. Schlenker and C. Escribie-Filippini, |
|
Inorg. Chem. 28, 1466 (1989). |
12.M.-H. Whangbo, R. Hoffmann and R. B. Woodward. Proc. R. Soc. London,
A366, 23 (1979).
13.J. Howell, A. Rossi, D. Wallace, K. Haraki and R. Hoffmann, QCPE, 4, 344
(1984).
14.M.-H. Whangbo , M. Evain, T. Hugbanks, M. Kertesz, S. Wijeysekera, C. Wilker, C. Zheng and R. Hoffmann, QCPE, 9, 591 (1989).
15.R. Hoffmann , J. Am. Chem. Soc. 112, 50 (1990).
16.L. Kihlborg, Arkiv Kemi, 21, 357 (1963).
17.G. Pacchioni, P. S. Bagus, M. R. Philpott and C. J. Nelin, Int. J. Quant. Chem.
XXXVIII, 675 (1990).
FROM CLUSTER TO INFINITE SOLID |
439 |
18.V. D. Sutula , A. P. Zeif, B. I. Popov and P. I. Vadash, React. Kinet. Catal. Letters ,9, 79 (1978).
19.A. B. El Awad, E. A. Hassan, A. A. Said and K. M. Abd El Salaam,
Monatschete für Chemie, 120, 199 (1989).
20.R. Hoffmann, J. Angew, Chem. Int. Ed. Engl. 26, 846 (1987).
21.L. E. Firment and A. Ferretti, Surf. Sci. 129, 155 (1983).
This page intentionally left blank.
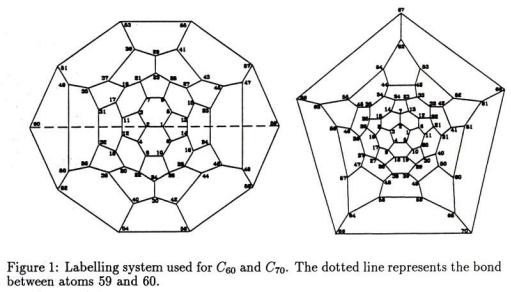
AB-INITIO CALCULATIONS ON MUONIUM ADDUCTS OF FULLERENES |
443 |
later. For the present it is sufficient to split these into three groups as indicated, using an obvious notation, in Fig. 2a.
Previous calculations on have indicated that the distortion to the cage in the neighbourhood of the point of attachment is so localised that it can be well represented by allowing the positions of only six carbon atoms to relax. It is assumed here that the same will apply to muonium adducts of and addition will take place according to process (1) at sites of unsaturation (Fig. 2a).
A typical group are atoms 1 through 6 in Fig. 1, forming a type a structure using a terminolgy introduced elsewhere [4]. A type a is identified as involving atoms from two pentagonal arrangements in the fullerene structure, the connecting bond (1-2) being short and presumably unsaturated. Three distinguishable type a structures
are illustrated for |
in Fig. 2b, the atoms in closed loops having the points of |
|||
muonium attachment indicated by |
and |
|
||
The groups of atoms within each closed loop is called a defect, the |
defect being |
|||
different from |
simply by |
the point |
of muonium attachment. Defect |
is at right |
angles to defects |
and |
From previous calculations [11] on |
the central |
two atoms of the defect are expected to |
change their hybridisation |
from |
with |
an inevitable distortion of the underlying fullerene cage. For example if the |
site |
||
is the point of muonation, the unpaired electron tends to localise on the |
site, |
||
each site changing its hybridisation from |
to approximately |
the associated |
distortion also affecting the four nearest neighbours. Note that all defects of type a contain at their centres one of the double bonds of Fig. 2a enabling the adduct to be formed according to process (1). Although can theoretically exist in one of five isomeric forms we have already identified three isomers of type a with an alkene type of unsaturation. The rest must be limited to regions of arene type unsaturation which form a band around the equator of Even so this gives rise to a further
AB-INITIO CALCULATIONS ON MUONIUM ADDUCTS OF FULLERENES |
445 |
2.2. RESONANCE THEORY
Resonance theory [15] contains essentially three assumptions beyond those of the valence bond method. Perhaps the most serious assumption is the contention that only unexcited canonical forms, non-polar valence bond structures or classical structures need be considered. Less serious, but no more than intuitive, is the proposition that the molecular geometry will take on that expected for the average of the classical structures. This is extended to the measurement of stability being greater the greater the number of classical structures. These concepts are still widely used in chemistry in very qualitative ways.
Molecules of the size of the fullerenes require such approaches to help rationalise the results. Even though the number of classical structures can be very large the same qualitative reasoning can be used as with the smaller molecules, typically benzene.
The enumeration of classical structures is easily accomplished using computers. It is simply a problem of determining how many ways a set of points (in our case carbon atoms) can be connected given rules governing their connectivity.
3.Results
All of the ab initio results are collected in Tables 2 and 3. The tables differ only in the theoretical method used. Table 2 used the ROHF method and Table 3 used the UHF method using optimised geometries from Table 2.
The numbering system of the carbon atoms used are given in Fig. 1 for
Further results from the ab initio calculations are collected in various figures where they are compared to the results of resonance theory. The results are displayed in Figs. 3, 4 and 5. The number of classical structures is compared with the UHFAA spin densities at each centre in Fig. 3. Correlations between the number of classical structures and spin density (Fig. 4) and bond length (Fig. 5) are plotted. The
calculated bond lengths in |
are rationalised using resonance theory in Table 4 from |
|
which a valence bond type structure of |
is suggested in Fig. 2a. The structures |