
Chemiluminescence in Analytical Chemistry
.pdf526 |
Popov and Lewin |
vance of environmental factors, life style, East-West gap, etc., on health, fitness for work and life-span)
Possible industrial applications include screening of substances with antiradical activity, quality testing of raw materials, pharmaceuticals, cosmetic products, fruit juices, wines, beers, edible oils, detection of food irradiation, and many more.
REFERENCES
1.G Lewin, I Popov. Entwicklung und experimentell-klinische Nutzung der Photo- chemolumineszenz-Methode fu¨r die Beurteilung der antioxidativen Homo¨ostase im Organismus. Berlin: Humboldt-Universita¨t, 1990.
2.G Lewin, I Popov. Med Hypoth 42:269–275, 1994.
3.NM Emanuel, YN Ljaskovskaja. Inhibition of Fat Oxidation. Moscow: Pistshepromisdat, 1961 (in Russian).
4.J Stocks, JMS Gutteridge, RJ Sharp, T Dormandy. Clin Sci 47:215–221, 1974.
5.J Frank, JV Geil, R Freaso. Food Technol 36:71, 1982.
6.DDM Wayner, GW Burton, KU Ingold, S Locke. FEBS Lett 187:33–37, 1985.
7.I Popov, J Ho¨rnig, R von Baehr. Z med Labor-Diagn 26:417–421, 1985.
8.E Niki, Y Yamamoto, Y Kamiya. Chem Lett 1267–1270, 1985.
9.GI Klebanov, IV Babenkova, YuO Teslkin, OS Komarov, YuA Vladimirov. Lab Delo 1:59–62, 1988 (in Russian).
10.NJ Miller, C Rice-Evans, MJ Davies, V Gopinathan, A Milner. Clin. Sci 84:407– 412, 1993.
11.M Nakano, T Ito, T Arimoto, Y Ushijima, K Kamiya. Biochem Biophys Res Commun 202:940–946, 1994.
12.A Ghiselli, M Serafini, G Maiani, E Azzini, A Ferro-Luzzi. Free Rad Biol Med 18: 29–36, 1995.
13.A Saramet, G Danila, I Paduraru, D Petrariu, R Olinescu. Arzneimittelforschung 46:501–504, 1996.
14.A Abella, C Messaoudi, D Laurent, D Marot, J Chalas, J Breux, C Claise, A Lindenbaum. Br J Clin Pharmacol 42:737–741, 1996.
15.P Palozza, C Luberto, GM Bartoli. Free Rad Biol Med 18:943–948, 1995.
16.MC Hanlon, DW Seybert. Free Rad Biol Med 23:712–719, 1997.
17.L Landi, D Fiorentini, M Lucarini, E Marchesi, GF Pedulli. Effect of the medium on the antioxidant activity of dipyridamole. In: SFRR Europe Summer Meeting, June 26–28, 1997, Abano Terme. Book of Abstracts, p 265.
18.E Niki. In: G Scott, ed. Atmospheric Oxidation and Antioxidants. Amsterdam: Elsevier, 1993, Vol III, pp 1–31.
19.B Halliwell, JMC Gutteridge. Arch Biochem Biophys 280:1–8, 1990.
20.SP Kochhar. In: G Scott, ed. Atmospheric Oxidation and Antioxidants. Amsterdam: Elsevier, 1995, Vol II, pp 71–131.
21.I Popov, P Falck, R von Baehr. Studia Biophys 115:39–44, 1986.
Photosensitized Chemiluminescence |
527 |
22.DV Magin, G Lewin, I Popov. Vopr Med Khim 45:70–79, 1999 (in Russian).
23.BA Rusin, In: B Tarusov, ed. Biochemiluminescence. Moscow: Nauka, 1983, pp 69–117 (in Russian).
24.G Merenyi, J Lind, TE Eriksen. J Am Chem Soc 108:7716–7726, 1986.
25.R Radi, TP Cosgrove, JS Beckman, BA Freeman. Biochem J 290 (Pt 1):51–57, 1993.
26.GN Semenkova, TM Novikova, SN Cherenkevich, AI Drapeza. Lab Delo 11:13– 15, 1991 (in Russian)
27.JM McCord, I Fridovich. J Biol Chem 244:6049–6055, 1969.
28.I Popov, G Lewin. J Biochem Biophys Methods 31:1–8, 1996.
29.M Ryan, L Grayson, DJ Clarke. Ann Clin Biochem 34(Pt 6):688–689, 1997.
30.A Sevanian, KJA Davies, P Hochstein. J Free Rad Biol Med 1:263–271, 1985.
31.K Bernhard. Helv Chim Acta 38:306–333, 1954.
32.CR Krishna Murthy. Proc Ind Nat Acad. Sci B48:163–175, 1982.
33.I Popov, G Lewin. In: L Packer, AN Glazer, eds. Methods in Enzymology. New York: Academic Press, 1999, Vol 300, pp 437–456.
34.G Lewin, I Popov. J Biochem Biophys Methods 28:277–282, 1994.
35.G Lewin, G Ku¨hn, U Deuse, M Bu¨hring, B Ramsauer, I Popov. Paradox of the antiradical capacity of blood plasma proteins. In: SFRR Europe Summer Meeting, July 2–5, 1999, Dresden, Germany. Book of Abstracts, p 029.
36.I Popov, G Lewin. Luminescence 14:1–6, 1999.
37.G Lewin, I Popov. Drug Res 44:604–607, 1994.
38.H Esterbauer, M Dieber-Rotheneder, G Waeg, G Striegl. Chem Res Toxicol 3:77– 92, 1990.
39.D Smith, VJ O’Leary, VM Darley-Usmar. Biochem Pharmacol 45:2195–2201, 1993.
40.H Esterbauer, M Dieber-Rotheneder, G Striegl, G Waeg. J Clin Nutr 53:314–321, 1991.
41.SR Maxwell, O Wiklund, G Bondjers. Atherosclerosis 111:79–89, 1994.
42.B Kalyanaraman, V Darley-Usmar, A Struck, N Hogg, S Parthasarathy. J Lipid Res 36:1037–1045, 1995.
43.RE Pacifici, KJA Devis. In: L Packer, AN Glazer, eds. Methods in Enzymology. New York: Academic Press, 1990, 186 B, pp 485–502.
44.ER Stadtman. Annu Rev Biochem 62:797–821, 1993.
45.I Popov, G Lewin. Free Rad Biol Med 17:267–271, 1994.

18
Application of Novel Acridan Esters as Chemiluminogenic Signal Reagents in Immunoassay
Gijsbert Zomer and Marjorie Jacquemijns
National Institute of Public Health and the Environment, Bilthoven, The Netherlands
1. |
INTRODUCTION |
530 |
|
|
1.1 |
Immunoassay |
531 |
|
1.2 |
Enzyme Immunoassay |
533 |
|
1.3 |
Practical Aspects of Enzyme Immunoassay |
533 |
2. |
CHEMILUMINOGENIC SIGNAL REAGENTS FOR HRP |
535 |
|
|
2.1 |
Enhanced Chemiluminescence Using Luminol and Analogs |
535 |
|
2.2 |
Enhanced Chemiluminescence Using Acridan Esters |
535 |
|
2.3 |
Mechanism of Enhanced Chemiluminescence |
536 |
|
2.4 |
Characteristics of the GZ-11 Acridan Ester Signal Reagent |
538 |
3. |
APPLICATIONS |
541 |
|
|
3.1 |
Atrazine Immunoassay |
541 |
|
3.2 |
Clenbuterol Immunoassay |
543 |
4. |
SYNTHESIS AND CHARACTERIZATION OF |
|
|
|
ACRIDAN ESTERS |
545 |
|
|
4.1 |
Acetanilide |
545 |
|
4.2 |
4-Chorophenylphenyl Amine |
545 |
|
4.3 |
2-Chloro-Acridine-9-Carboxylic Acid |
546 |
This chapter is dedicated to the memory of J. F. C. (Hans) Stavenuiter, a long-term friend and mentor.
529
530 |
Zomer and Jacquemijns |
|
4.4 |
Perfluoro-t-Butyl 2-Chloroacridine-9-Carboxylate |
546 |
4.5 |
Perfluoro-t-Butyl 2-Chloro-10-Methylacridinium-9-Carboxylate |
|
|
Trifluoromethanesulfonate |
547 |
4.6 |
Perfluoro-t-Butyl 2-Chloro-10-Methylacridane-9-Carboxylate |
|
|
(GZ-11) |
547 |
1. INTRODUCTION
Bioluminescence, the phenomenon of biological light emission, has fascinated mankind for many centuries. Scientists have been intrigued by it, and for many years have tried to answer ‘‘simple’’ questions like how and why certain animals and bacteria bioluminesce. This research has led to new insights in (molecular) biology and biochemistry. For example, in the 1960s, McCapra studied the chemical mechanisms of bioluminescence and devised a model for firefly luciferin: the acridan esters (Fig. 1) [1–5].
In the firefly, the carboxyl group of luciferin is activated. Key features of both compounds include an easily autoxidizable CH group, an activated carbonyl group in juxtaposition, and an oxidation product that is very fluorescent. Studies of the chemistry of the model acridan ester have led to a better understanding of firefly bioluminescence including correct prediction of the structure of the oxidized luciferin product.
One of the spinoffs of this work has been the appreciation that bioor chemiluminescence could be used as an aid in analytical chemistry. This has led to the successful applications of acridinum esters as labels in immunoassays [6] and DNA probes [7, 8].
Using acridinium esters (Fig. 2) as chemiluminogenic labels, very sensitive immunoassays were developed [6]. Also commercially, immunoassays using acridinium ester labels have proven to be successful. The sensitivity of detection
Figure 1 Structures of firefly luciferin and acridan ester.
Novel Acridan Esters |
531 |
Figure 2 Structure of acridinium ester.
of acridinium ester chemiluminescence is in the order of 10 amol. This is considerably better than the sensitivity of radioactive labels like 3H and 125I. Even greater sensitivities can be reached by using an enzyme as the label: in this case, because of enzyme turnover, many chemiluminescent molecules can be produced from one enzyme molecule. Examples include the luminol-enhanced chemiluminescence [9–11] catalyzed by horseradish peroxidase (HRP) and certain luminol analogs [12] reported to be more efficient than luminol.
Recently, it was found that certain acridan esters could be oxidized to acridinium esters in a reaction catalyzed by HRP [13, 14]. Like the luminol case it was found that the light yield of the reaction could be increased when certain additives (enhancers) were added.
1.1 Immunoassay
Immunoassays are based on the ability of the immune system to produce a virtually unlimited variety of antibodies each with a high affinity for foreign compounds (immunogens like viruses, bacteria, proteins, and haptens). Analytically, this phenomenon can be exploited by detection of this immunoreaction using labeled antibodies or antigens (i.e., compounds that can be bound by antibodies). The equilibrium between antibody (Ab), antigen (Ag), and immune complex (AbAg) may be expressed as:
ka |
|
Ab Ag i Ab Ag |
(1) |
kd
where ka and kd represent the association and dissociation rate constants, respectively. The equilibrium (affinity) constant K is defined as:
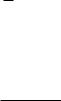
532 |
Zomer and Jacquemijns |
K ka kd
And, according to the law of mass action:
ka[Ab][Ag] kd[Ab Ag]
This becomes:
k [Ab Ag]
[Ab] [Ag]
In a so-called competitive immunoassay format the antigen competes with a labeled antigen for a limited number of antibody-binding sites. It can be shown that in this case the ultimate sensitivity of the assay (when the [Ab] approaches zero) is dependent on the equilibrium constant K and the reliability of the signal measurement of the bound fraction at zero dose [15].
An example of an immunoassay format is shown in Figure 3. This immunoassay format relies on partial saturation of the solid-phase antibody (Ab) by the antigen (Ag) and on its competition with the labeled antigen (Ag-L) for the available antibody sites. At low antibody and tracer concentrations the sensitivity of
Figure 3 Layout of competitive solid-phase antibody immunoassay format; in an incubation step antigen (Ag) and labeled antigen (Ag-L) compete for the solid-phase antibodybinding sites (Ab); after the solid-phase antibodies are washed, the activity of the bound labeled antigen is measured, and a calibration curve constructed. The measured signal is inversely proportional to the antigen concentration.
Novel Acridan Esters |
533 |
this type of assay increases since at low concentrations a variation in the amount of competing antigen has a larger influence on the change of signal that is ultimately measured. When using low concentrations of antibody and tracer, the rate of immunocomplex formation is reduced, resulting in relatively long incubation times. It can be shown that in this case the ultimate sensitivity of the assay (when the [Ab] approaches zero) is dependent on the equilibrium constant K and the reliability of the signal measurement of the bound fraction at zero dose (B0) [15]. For example, an antibody-antigen system with a K of 1010 M 1 and a relative error of 5% can be detected at 5* 10 12 M. With a low-molecular-weight compound (mw 200) this corresponds to a maximal attainable sensitivity of 10 9 g/L or 1 ppt.
1.2 Enzyme Immunoassay
1.2.1Introduction
Using enzymes as labels in immunoassays potentially affords much more sensitive tracer (i.e., enzyme-labeled antigen or antibody) detection in comparison with radiolabels because of the great catalytic power resulting in generation of many product molecules from one enzyme molecule through turnover. A major advantage of radiolabels is that their measurement is not prone to chemical interference. This is not the case with enzymes. Measurement of enzyme activity is strongly influenced by suboptimal temperature, pH, salt concentration, etc. However, the ease and low price of enzyme detection, together with the increased costs of using radiolabels, has made enzymes popular labels in immunoassay. The most widely used enzyme for immunoassay purposes is horseradish peroxidase (HRP)
1.2.2Enzyme Detection
Although HRP is often detected and quantified using a chromogenic substrate (actually, hydrogen peroxide is the substrate for HRP, generating an oxidized form of HRP, which is subsequently reduced in two steps converting a hydrogen donor DH to an oxidized donor D; in this case the chromogenic substrate is colorless DH, which is transformed to colored product D), there are other possibilities to measure HRP. One of the most sensitive and convenient is use of chemiluminogenic substrates.
1.3 Practical Aspects of Enzyme Immunoassay
An enzyme-linked immunosorbent assay (ELISA) has two major components. The first is the immunological reaction that occurs between an antigen and antibody. This reaction is crucial and needs careful optimization. The second compo-
534 |
Zomer and Jacquemijns |
nent is the surface to which antigens or antibodies are immobilized. This aspect too needs consideration with respect to the effect of the solid phase on, e.g., reproducibility of antigen or antibody coating, nonspecific binding, sensitivity of the assay, etc. Furthermore, parameters like temperature, pH, composition of coating and assay buffer, incubation times, etc., play important roles with respect to assay sensitivity and reliability. In the next sections some of these factors are discussed in detail, taking as an example the assay format described in Figure 3.
1.3.1Coating of the Solid-Phase with Antibody
Although other assay containers can be used (e.g., coated tubes or beads), the most popular one is the 96-well microtiter plate. ELISAs that are detected in a microplate luminometer must be performed in opaque white or black plates.
Biomolecules like antibodies attach to surfaces via a variety of mechanisms. This attachment phenomenon is controlled by the chemical properties of the surface, but can be influenced by factors such as pH and temperature. In the case of antibody coating to a solid support the use of so-called medium-binding plates is to be recommended. Coating conditions can be optimized by performing a checkerboard titration (in the following example the optimal coating antibody concentration is determined):
A.Antibody is serially diluted across the plate (starting concentration of approximately 10 g/mL in coating buffer (PBS or carbonate buffer), allowed to coat to the surface (overnight at 4°C), and excess is washed away.
B.The surface is then blocked (PBS containing 0.02% Tween 20 and 1% BSA).
C.The antigen is serially diluted down the plate, allowed to react with the immobilized antibody, and excess is washed away (PBS containg 0.1% Tween 20, PBST).
D.Enzyme-labeled antigen is used at a constant (and excess) concentration of approximately 10 g/mL (such that it is not the limiting factor in the assay), allowed to react, and excess is washed away (PBST).
E.Signal reagent is added to each well.
F.Following signal detection, select the optimal reading (this reading is normally chosen based on the following: readings will increase from the bottom right corner to the upper left corner of the plate; the optimal reading lies just prior to the set of wells exhibiting the highest readings).
G.From the well corresponding to the optimal reading, derive the optimal concentration of coating antibody.
Novel Acridan Esters |
535 |
This procedure can be repeated for other coating conditions (time, temperature, pH, buffer compositions, blocking steps, wash buffers, etc.).
1.3.2Determining the Optimal Concentration of Labeled Antigen
The optimal concentration of labeled antigen can also be determined via a checkerboard titration protocol:
A.The antigen is serially diluted down the plate (optimized with respect to antibody concentration as described in Sec. 1.3.1.), allowed to react with the immobilized antibody, and excess is washed away (PBS containing 0.1% Tween 20, PBST).
B.Enzyme-labeled antigen is serially diluted across the plate, allowed to react, and excess is washed away (PBST).
C.Signal reagent is added to each well.
D.Following signal detection, select the optimal set of column wells (this will be dependent on the goal of the assay: if, for instance, a screening assay is required with a certain cutoff concentration, e.g., 0.1 ng/mL for pesticides in water, then the labeled antigen concentration that yields a B/B0 of 50% at 0.1 ng/mL will be ideal).
E.From the wells corresponding to the optimal reading, derive the optimal concentration of labeled antigen.
2. CHEMILUMINOGENIC SIGNAL REAGENTS FOR HRP
2.1Enhanced Chemiluminescence Using Luminol and Analogs
In the early 1980s a serendipitous discovery was made when to a mixture of HRP, luminol, and hydrogen peroxide in buffer, firefly luciferin was accidentally added. The light yield of the HRP-catalyzed peroxidation of luminol was greatly enhanced. This remarkable discovery marked the beginning of a very successful analytical tool for immunoassay and all kinds of blotting (protein, DNA, and RNA) applications [16–18].
2.2 Enhanced Chemiluminescence Using Acridan Esters
In 1994 at the 8th International Symposium on Bioluminescence and Chemiluminescence, Schaap and Akhavan-Tafti presented a new technology for the detection of HRP [13, 19, 20]. They proposed the use of certain aromatic acridan esters (see Fig. 4 for the structures) as part of a signal reagent for very sensitive detection