
5 курс / Пульмонология и фтизиатрия / Clinical_Manifestations_and_Assessment_of_Respiratory
.pdf
c. 1250
d. >3000
3.Which of the following are the major anatomic alterations of the lungs associated with near drowning victims? 1. Consolidation
2.Bronchospasm
3.Increased alveolar-capillary membrane thickness
4.Atelectasis
5.Excessive bronchial secretions
a.3 and 5 only
b.2 and 4 only
c.3, 4, and 5 only
d.1, 2, 3, 4, and 5
4.Which of the following clinical manifestations are associated with near drowning victims? 1. Frothy, pink sputum
2. Crackles
3. Increased pH
4. Increased
a.1 and 2 only
b.3 and 4 only
c.2, 3, and 4 only
d.1, 2, 3, and 4 only
5.Which of the following pulmonary function testing values are associated with near drowning victims? 1. N or ↓ FEVT
2.↓ FVC
3.↓ RV
4.N or ↑ FEV1/FVC ratio
a.1 and 2 only
b.3 and 4 only
c.2, 3, and 4 only
d.1, 2, 3, and 4
1It has long been known that mechanical ventilation can produce a variety of lung injuries referred to as ventilator-induced lung injury (VILI), pulmonary volutrauma, and pulmonary barotrauma. VILI stress fractures of the pulmonary capillary endothelium, epithelium, basement membrane, and in severe cases lung rupture. Lung ruptures can lead to leakage of fluid, protein, and blood into tissue and air spaces or leakage of air into tissue spaces. This condition can be followed by an inflammatory response and possibly a reduced defense against infection. Pulmonary volutrauma is damage to the lung caused by overdistention by a mechanical ventilator set for an excessively high tidal volume. Pulmonary barotrauma is damage to the lungs caused by rapid or extreme pressures generated by mechanical ventilation. Predisposing factors for VILI, pulmonary volutrauma, and pulmonary barotrauma include (1) mechanical ventilation with high peak inspiratory volumes and pressures, (2) mechanical ventilation with a high mean airway pressure, (3) structural immaturity of lung and chest wall, (4) surfactant insufficiency or inactivation, and (5) preexisting lung disease (see Chapter 11).
2Permissive hypercapnia: Mechanical ventilation was traditionally applied with the goal of normalizing arterial blood gas values, particularly the arterial carbon dioxide tension (PaCO2). However, this is no longer the primary objective of
mechanical ventilation. Today, the emphasis is on maintaining adequate gas exchange while—and, importantly—minimizing the risks of mechanical ventilation. Common strategies used to reduce the risk for mechanical ventilation include (1) low tidal volume ventilation to protect the lung from ventilator-associated lung injury in patients with acute lung injury (e.g., ARDS) and (2) reduction of the tidal volume, respiratory rate, or both to minimize intrinsic positive end-expiratory pressure (i.e., auto-PEEP) in patients with obstructive lung disease (e.g., COPD). Although these mechanical ventilation strategies may result in an increased PaCO2 level (hypercapnia), they do help protect the lung from barotrauma (i.e., physical damage
to lung tissues caused by excessive gas pressures). The lenient acceptance of the hypercapnia is called permissive hypercapnia. In most cases the patient's PaCO2 is adequately maintained by an increased ventilatory rate that offsets the
decreased tidal volume. The PaCO2, however, should not be permitted to increase to the point of severe acidosis. The most current consensus suggests it is safe to allow pH to fall to at least 7.20 (http://www.ARDSNet) (see Chapter 11).
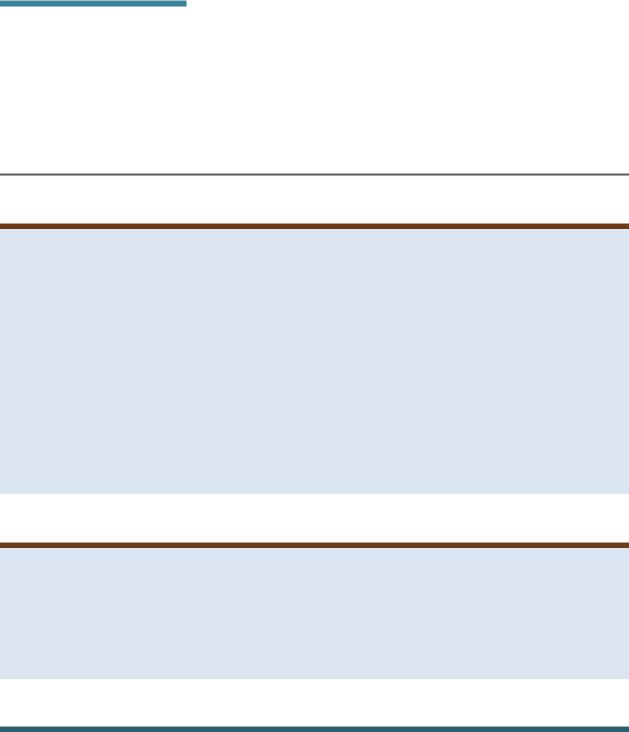

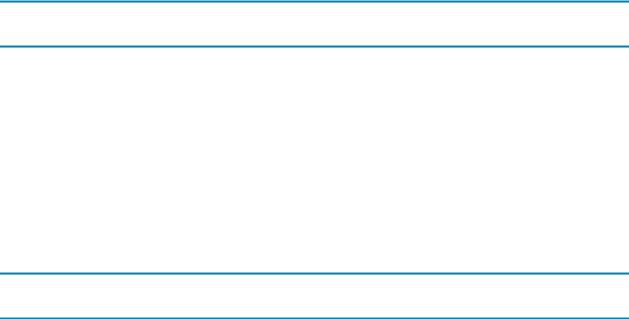
TABLE 45.1
The Approximate Percentage of Body Surface Area (BSA) for Various Body Regions of Adults and Infants
Anatomic Region |
BSA in Adults (%) |
BSA in Infants (%) |
Entire head and neck |
9 |
18 |
Each arm |
9 |
9 |
Anterior trunk |
18 |
18 |
Posterior trunk |
18 |
18 |
Genitalia |
1 |
1 |
Each leg |
18 |
13.5 |
NOTE: The “rule of nines” is used to estimate percentage of injury; each of the areas listed here represents about 9% or 18% of the body surface area. This rule does not apply to infants' legs.
Smoke can result from either pyrolysis (smoldering in a low-oxygen environment) or combustion (burning, with visible flame, in an adequate-oxygen environment). Smoke is composed of a complex mixture of particulates, toxic gases, and vapors. The composition of smoke varies according to the chemical makeup of the material that is burning and the amount of oxygen being consumed by the fire. Table 45.2 lists some of the more common toxic substances produced by burning products that are frequently found in office, industrial, and residential buildings.
TABLE 45.2
Toxic Substances and Sources Commonly Associated With Fire and Smoke
Substance |
Source |
Aldehydes (acrolein, acetaldehyde, formaldehyde) |
Wood, cotton, paper |
Organic acids (acetic and formic acids) |
|
Carbon monoxide, hydrogen chloride, phosgene |
Polyvinylchloride |
Hydrogen cyanide, isocyanate |
Polyurethanes |
Hydrogen fluoride, hydrogen bromide |
Fluorinated resins |
Ammonia |
Melamine resins |
Oxides of nitrogen |
Nitrocellulose film, fabrics |
Benzene |
Petroleum products |
Carbon monoxide, carbon dioxide |
Organic material |
Sulfur dioxide |
Sulfur-containing compounds |
Hydrogen chloride |
Fertilizer, textiles, rubber manufacturing |
Chlorine |
Swimming pool water |
Ozone |
Welding fumes |
Hydrogen sulfide |
Metal works, chemical manufacturing |
Although in some instances the toxic components of the smoke may be obvious, in most cases the precise identification of the inhaled toxins is not feasible. In general, the inhalation of smoke with toxic agents that have high water solubility (e.g., ammonia, sulfur dioxide, and hydrogen fluoride) affects the structures of the upper airway. In contrast, the inhalation of toxic agents that have low water solubility (e.g., hydrogen chloride, chlorine, phosgene, and oxides of nitrogen) affects the distal airways and alveoli. Many of the substances in smoke are caustic and can cause significant injury to the tracheobronchial tree (e.g., aldehydes [especially acrolein], hydrochloride, and oxides of sulfur).
Body Surface Burns
Because the amount and severity of body surface burns play a major role in the patient's risk for mortality and morbidity, an approximate estimate of the percentage of the body surface area burned is important. Table 45.1 lists the approximate percentage of surface area for various body regions of adults and infants. The severity and depth of burns are usually defined as follows:
•First-degree burn (minimal depth in skin): Superficial burn, damage limited to the outer layer of epidermis. This burn is characterized by reddened skin, tenderness, and pain. Blisters are not present. Healing time is about 6 to 10 days. The result of healing is normal skin.
•Second-degree burn (superficial to deep thickness of skin): Burns in which damage extends through the epidermis and into the dermis but is not of sufficient extent to interfere with regeneration of epidermis. If secondary infection results, the damage from a second-degree burn may be equivalent to that of a third-degree burn. Blisters are usually present. Healing time is 7 to 21 days. The result of healing ranges from normal to hairless and depigmented skin with a texture that is normal, pitted, flat, or shiny.
•Third-degree burn (full thickness of skin including tissue beneath skin): Burns in which both epidermis and dermis are destroyed, with damage extending into underlying tissues. Tissue may be charred or coagulated. Healing may occur after 21 days or may never occur without skin grafting if the burned area is large. The resultant damage heals with hypertrophic scars (keloids), chronic granulation, and contractures.
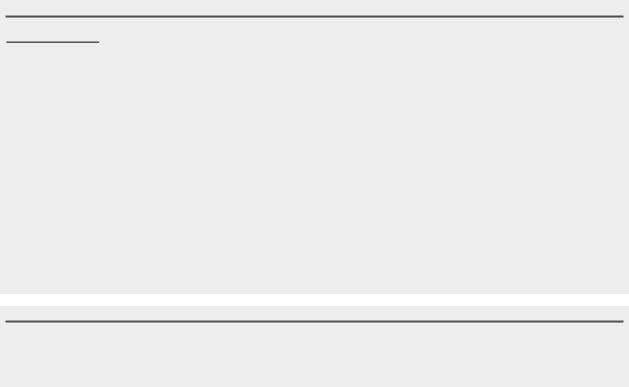

Acute Alveolar Hyperventilation With Hypoxemia2 (Acute Respiratory Alkalosis)
pH |
PaCO2 |
|
PaO2 |
SaO2 or SpO2 |
|
|
|
|
|
↑ |
↓ |
↓ |
↓ |
↓ |
|
|
(but normal) |
or normal |
or normal |
2See Table 5.5 and related discussion for the acute pH, PaCO2, and changes associated with acute alveolar hyperventilation.
Severe Smoke Inhalation and Burns With Metabolic Acidosis
COHb |
pH3 |
PaCO24 |
|
3 |
PaO2 |
SaO2 or SpO2 |
↑ |
↓ |
↓ |
↓ |
|
↓ |
↓ |
|
(lactic acidemia) |
|
|
|
or normal (but tissue hypoxemia is present) |
or normal |
3When tissue hypoxia is severe enough to produce lactic acid, the pH and values will be lower than expected for a particular PaCO2 level.
4In severe burns with ARDS the PaCO2 may be elevated and combined respiratory and metabolic acidosis may be present.
COHb, Carboxyhemoglobin.
When carbon monoxide (CO) or cyanide poisoning is present, the pH may be decreased during the early stages of smoke inhalation. This decrease in pH occurs because patients with severe CO or cyanide poisoning commonly have lactic acidosis as a result of tissue hypoxia, even in the presence of a normal PaO2. Therefore when CO or cyanide poisoning is
present, the patient may demonstrate the following arterial blood gas values.
Severe Smoke Inhalation and Burns With Respiratory and Metabolic Acidosis
Acute Ventilatory Failure (Acute Respiratory Acidosis) and Metabolic Acidosis
COHb |
pH5 |
PaCO 6 |
5 |
PaO |
2 |
SaO |
2 |
or SpO |
2 |
|
|
2 |
|
|
|
||||
|
|
|
|
|
|
|
|
|
|
↑ |
↓ |
↑ |
↓ |
↓ |
|
↓ |
|
|
|
|
|
|
|
|
|
or normal |
5When tissue hypoxia is severe enough to produce lactic acid, the pH and values will be lower than expected for a particular PaCO2 level.
6In severe burns with ARDS the PaCO2 may be elevated and combined respiratory and metabolic acidosis may be present.
COHb, Carboxyhemoglobin.
Oxygenation Indices
Smoke Inhalation and Burns
|
Early and Intermediate Stages |
Late Stage |
DO2 |
↓ |
↓ |
VO2 |
↑ |
↓ |
|
↑ |
↓ |
|
|
|
O2ER |
↑ |
↓ |
|
↓ |
↓ |
|
|
|
When CO or cyanide poisoning is present, the oxygenation indices are unreliable because the PaO2 is often normal in the
presence of carbon monoxide poisoning, and when cyanide poisoning is present, the tissue cells are prevented from consuming oxygen. Both of these conditions cause falsely high pulse oximetry readings. For example, when CO is present, a normal DO2 value may be calculated when, in reality, the patient's oxygen transport status is extremely low. When
cyanide poisoning is present, the patient's VO2 may appear normal or increased, when in actuality the tissue cells are
extremely hypoxic. Typically these problems are not present during the intermediate and late stages in the presence of appropriate treatment—that is, once the carbon dioxide and/or cyanide has been removed.
Hemodynamic Indices
Cardiogenic Pulmonary Edema7

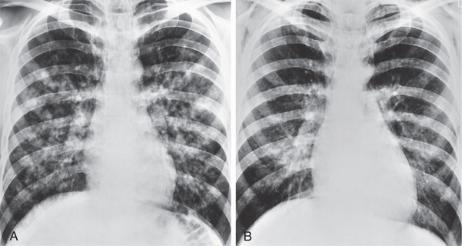