
книги / Структурно-механические свойства эластомерных композитных материалов
..pdf
Thus, the molecular graph allows one to estimate the interstitial molecular weight Мs of a cross-linked elastomer, which is repeated ∞ times and is included in the mathematical description of the structural-mechanical properties of a filled elastomer. Assuming that R3 << R2 and R1, the parameter Мs can be esti-
mated as a quantity proportional to the increment of the molar fraction of linear polymerization – ( R1 f12 R2 )n – according to the molecular graph.
In [2], about hundred empirical formulas for the concentration dependence of the factor of dynamic viscosity η of suspensions were analyzed. Later, in [3], on an experimental basis, a formula currently widely used was suggested:
Rf |
f |
|
Ef |
|
|
/ |
m |
2 |
(8) |
|
|
|
|
1 |
1,25 |
|
|
, |
|||
0 |
E0 |
|
|
|||||||
|
|
|
|
1 / m |
|
|
whose validity for polymer compositions was substantiated in [4-18]. Here, φ is the volume fraction of filler; ϕm is the limiting volume fraction of filler, depending on the form and fractional composition of filler particles and on the physical and chemical interaction at the polymer binder–filler interface; the subscripts “f” and “0” mean the filled and free states of the elastomer. The value of ϕm can be determined by viscosimetry [19] or calculated by the com- binatorial-multiplicative method [20], with account of the volume fraction of the polymeric binder immobilized by particles of a particular filler.
The parameter φ/φm has the meaning of the volume degree of effective filling of the binder.
Expression (8) allows us to reduce formula (7) to the form required for a filled elastomer
1/3 |
|
|
|
|
0,225 |
10 |
3 |
(T Tg ) |
2 |
|
1 |
|
1 |
|
|
|
|
|||||||||
ch r |
RT 1 29exp |
|
|
|
|
a |
|
|
|
|||||||||||||||||
|
|
|
|
|
|
|
/ m |
|
|
2 |
|
|
|
|
|
|
|
|
|
|
|
|
(9) |
|||
|
|
|
|
|
|
( 2 ). |
|
|
|
|
|
|
|
|
|
|
||||||||||
|
|
|
1 |
1,25 |
|
|
|
|
|
|
|
|
|
|
|
|
|
|||||||||
|
|
|
|
|
|
|
|
|
|
|
|
|
|
|
|
|
|
|||||||||
|
|
|
|
|
1 / m |
|
|
|
|
|
|
|
|
|
|
|
|
|
|
|||||||
From Eq. (9), we can also derive an expression for the initial |
a 1 |
=1 vis- |
||||||||||||||||||||||||
|
|
|
|
|
|
|
|
|
|
|
|
|
|
|
|
|
|
|
|
|
|
|
|
|
|
|
coelastic modulus of a composition: |
|
|
|
|
|
|
|
|
|
|
|
|
|
|
|
|
|
|
|
|
||||||
d |
|
|
1/3 |
RT 1 29exp |
|
|
|
|
3 |
|
|
|
|
|
2 |
1 |
|
|||||||||
|
|
|
|
|
|
|
|
|
||||||||||||||||||
E d |
|
1 3 ch r |
0,225 10 |
|
(T Tg ) |
a |
|
|||||||||||||||||||
|
|
|
||||||||||||||||||||||||
|
|
|
|
|
|
|
|
|
|
/ m |
2 |
|
|
|
|
|
|
|
|
|
|
(10) |
||||
|
|
|
|
1 |
1,25 |
|
|
. |
|
|
|
|
|
|
|
|
|
|
|
|||||||
|
|
|
|
1 |
/ m |
|
|
|
|
|
|
|
|
|
|
|
||||||||||
|
|
|
|
|
|
|
|
|
|
|
|
|
|
|
|
|
|
|
|
|
11
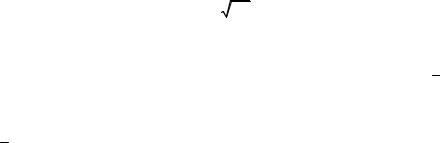
For describing the structural-mechanical situation in the case of possible delamination of binder from the surface of filler particles [4], Eq. (9) is transformed to the form
ch r1/3RT 1 29exp 0,225 10 3 (T Tg )2 1a 1
|
|
/ |
|
n |
|
1 |
|
|
2 |
(11) |
||
|
|
m |
1 |
|
|
exp( 0,5ti2 )dti |
|
( 2 ), |
||||
1 |
1,25 |
|
|
|
i |
|||||||
1 / m |
2 |
|||||||||||
|
|
i 1 |
|
|
|
|
|
|||||
|
|
|
|
|
|
|
1 |
|
|
where the kinetics of accumulation of delaminations of binder from filler particles is described by the Gaussian function; n is the number of volume parts φi
of delaminated fractions (types) of disperse filler; ti ( i0,5) / i is a pa-
rameter of the function, αi05 – is the elongation of a specimen corresponding to half the volume of delaminated particles of an ith fraction (type) of filler, andi is the root-mean-square scattering for the i th fraction (type) of filler. The
quantity αi05. determines the beginning of delamination, and σi is the rate of the process of disruption of solidity of the material.
The effect of delamination of polymeric binder from the surface of filler particles is reflected by the “hump-shaped” form of tension diagrams. The tests carried out on transparent flat models of elastomeric binders containing silica particles of different dispersity made it possible to reveal, by means of synchronous filming, the kinetics of the process of accumulation of particles delaminated from binders of various polarity (Fig. 4). The volume fraction of filler was 0.3. From data of the figure, it is seen that the process of accumulation can be well described by means of the normal distribution function, reflecting changes in the reinforcement factor Rf (for an ith fraction or type of filler) during tension of a specimen:
|
|
|
|
|
/ m |
n |
|
|
|
|
2 |
|
|
|
|
|
|
|
|||||
|
f |
|
|
|
|
|
i |
|
i |
|
|
R |
|
|
1 |
1,25 |
|
1 |
F |
( ) |
|
|
, |
|
|
|
|
|
1 / m i 1 |
|
|
|
|
|
where Fi ( ) 1/ 2 exp( ti2 / 2)dti is the Gaussian function of normal distri-
1
bution for the ith fraction or type of filler. It is also seen that a less polar binder (polybutylisoprene) starts to separate earlier than the more polar one (polydiene epoxy urethane). It is noticeable that, all other things being equal, larger particles separate earlier than finely dispersed ones.
12
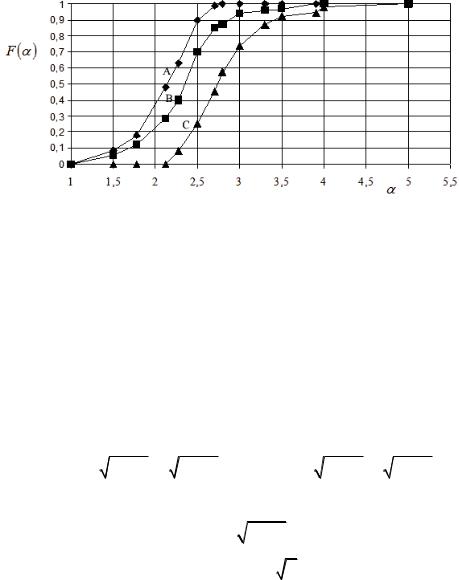
Fig. 4. Kinetics of the delamination process of silica particles from elastomers based on polybutyl isoprene (1, 2) and polydiene epoxy urethane (3). Filler fractions: 1400-1600 (1, 3) and 450-550 μm (2).
An analysis of the curves showed that the distribution parameter ti had the following values:
tA ( 1,7) / 0,5, |
tB ( 2,0) / 0,8, |
tC ( 3,1) / 0,5. |
The limiting mechanical characteristics( b , b ) in the conditions of
uniaxial tension of a filled elastomer can be estimated by taking into account the rate and magnitude of deformation of the average -statistical layer of polymeric binder between the solid particles of filler:
|
f |
|
o |
(1 3 / ) 3 |
/ ; |
b b (1 3 |
/ ) 3 |
/ |
m |
, |
||
|
|
m |
m |
f |
o |
|
m |
|
|
|||
In terms of breaking strains, we have |
|
|
|
|
|
|
||||||
|
|
|
|
b b (1 3 / |
m |
). |
|
|
|
(12) |
||
|
|
|
|
f |
o |
|
|
|
|
|
|
Contrary to the dependence bf ob (1 3 ), suggested in [4], formula
(12) well describes experimental data if the interfacial delamination of a filled elastomer in tension is practically absent.
In the case of “hump-shaped” tension diagrams, as the limiting characteristics, we accept their values at the point of “maximum”, but in the presence of a “plateau” – at its midpoint. In such a way, operating life of a material is determined.
Figure 5 shows a generalized relation between the breaking strain( ob ) of free elastomers on different polymeric bases and the effective concentration
13
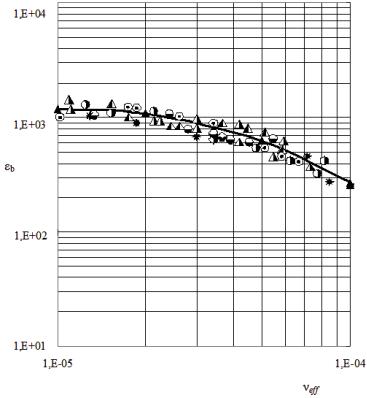
νeff of transverse bonds. We tested the transversely cross-linked polyesterurethane, polybutadiene, polybutylisoprene, and polydiene epoxy urethane, as well as these materials partially plasticized by dibutyl phosphate, dioctyl sebacate, and transformer oil. The data are presented for Т = 293 K.
It is seen that the maximum level of limiting strain( ob 1000 % ; ob 11 )
is ensured at an effective concentration of transverse bonds in the range of (1-2)·10–5 mol/cm3 at the expense of the decreased part of “physical” components of the transverse network. A further rarefaction of the transverse chemical network in similar polymer systems is restricted by the increasing “defectness” of the systems upon their three-dimensional polymerization.
Fig. 5. Experimental relationship b0 f eff for various polymer binders
based on ▲ – polysulfide, ♦ – polyesterurethane, ○ – polyisoprene divinyl, ◊ – polybutadiene, polybutylisoprene, and ■ – polydiene epoxy urethane at Т = 293 K an d = 1.2·10–3 s–1
14
ENGINEERING APPLICATION
The developed physical and mathematical description of the relation between the mechanical characteristics of a filled elastomer and the basic structural parameters of a composition makes it possible to solve two engineering
problems. |
|
|
|
|
|
The |
|
direct |
problem: by using assigned |
structural |
parameters |
(( ch , r , |
Tg , / m ) of a composition, test temperature Т, and deformation |
||||
rate (a 1) , |
predict |
the dependence f ( ) and |
the initial |
viscoelastic |
|
|
|
|
|
|
|
modulus Е.
The inverse problem: according to desired mechanical characteristics [σ(α),E] at required test temperatures Т and strain rate (T, -1) , find the basic
structural parameters νch, φr and Tg, φ/φm of a composition.
The computer program developed includes formulas (9)-(12) in combination with the calibration dependence ob f ( eff ) (see Fig. 4).
In the case of engineering solution of the direct problem, the lowmolecular rubbers – polydiene epoxy urethane of trademark PDI-3B with epoxy end groups and polybutadiene of trademark SKD-KTR with carboxyl end groups – were used. Their three-dimensional cross-linking was performed with the help of the three-functional epoxy resin of trademark EET-1. The polymeric binder contained dibutyl phthalate [φsw = (1-φr) = 0.3]. as a softener. The filler was silicon dioxide in the form of a mixture of large (12001500 μm), medium-size (150-180 μm), and fine (0.5-1.5 μm) fractions in the ratio 50:30:20, respectively. The necessary thermal and physical parameters of the constituents were taken from a handbook. The “freedom” of delamination was assumed only for the large fraction of filler, according to the data given in Fig. 4. The calculated and experimental tension diagrams were obtained at a temperature of 293 K and a standard strain rate 1.2·10-3s-1. Figure 6 demonstrates a satisfactory agreement between the predicted and experimental data in this problem.
For solving the inverse problem, the same constituents were used. Table presents the technical requirements to the mechanical characteristics of a filled elastomer created for the coverings of sports constructions.
As a result of processing the data of computer program, a composition with the following parameters was designed:
- the glass-transition temperature of polymer binder – 185 K;
15

-the volume fraction of polymer in the binder – 0.60;
-the volume fraction of softener in the binder – 0.40;
-the volume fraction of filler – 0.83 at φm = 0.96;
-the concentration of transverse chemical bonds in the polymer – 2·10-5 mol/cm3;
-the volume parts of filler fractions – (1200-1500 μm): (150-180 μm): (0.5-1.5 μm) = 0.591 : 0.279 : 0.130, respectively;
- the parameter |
of delamination |
of binder |
from the large fraction |
|
|
|
tcr ( 1,25) / 0,7. |
|
|
|
|
|
|
Required Mechanical Characteristics of a Filled Elastomer |
|
|
||||
|
|
|
|
|
|
|
Characteristics |
Temperature, K |
|
|
323 |
|
|
|
223 |
273 |
|
|
|
|
Initial viscoelastic |
60.1 |
14.8 |
|
3.7 |
|
|
modulus, MPa |
|
|
|
0.69 |
|
|
Maximum stress, MPa |
3.47 |
1.47 |
|
|
|
|
Strain at the maximum |
24.4 |
36.4 |
|
39.6 |
|
|
stress, % |
|
|
|
0.66 |
|
|
Breaking stress, MPa |
3.37 |
1.44 |
|
|
|
|
Breaking strain, % |
27.9 |
43.0 |
|
46.7 |
|
|
Thus, the results of the physical and mathematical investigations performed and the engineering applications considered can be recommended for reducing the time of design and the consumption of materials in creating promising polymer composites.
σ, MPa
α
Fig. 6. Conditional stress σ vs elongation α : continuous line – calculation and dots – experiment
16

σ, MPa |
4 |
|
|
|
|
1 |
|
|
3,5 |
|
|
|
|
|
|
|
|
|
|
|
|
|
|
|
3 |
|
|
|
|
|
|
|
2,5 |
|
|
|
|
|
|
|
2 |
Е |
|
|
|
|
2 |
|
1,5 |
|
|
|
|
|
|
|
1 |
|
Е |
|
|
|
3 |
|
|
|
|
|
|
||
|
|
|
|
|
|
|
|
|
0,5 |
α |
Е |
|
|
|
|
|
0 |
|
|
|
|
|
|
|
|
0 |
10 |
20 |
30 |
40 |
ε,% 50 |
Fig. 7. Calculated and required relations between the stress σ and strain ε (%) for filled elastomer at T = 223 (1), 273 (2), and 323 K (3) and wanted values of the initial viscoelastic modulus Е0; ○ – maximum stress and ● – breaking stress.
CONCLUSIONS
In the present study, a physical and mathematical description of the relation between the mechanical characteristics of a filled elastomer and the basic parameters of the composition is developed, and examples are presented for engineering solutions to the direct and inverse problems by creating new polymer composite materials with required properties.
REFERENCES
1.Treloar I.R.G. “The elasticity and related properties of rubbers,” Rubber Rew., 47, 625-696 (1974).
2.Rutgers J.R. Rheological Acta, 2, No. 4, 305-348 (1962).
3.Chong J.S., Christiansen E.B., and Boer A.D. J. Appl. Polym. Sci., 15, 2007-2024 (1971).
4.Nielsen L.E. Mechanical Properties of Polymers and Composites, Marcel Dekker, Inc., New York (1974).
5.Bueche F. Physical Properties of Polymers, Wiley Interscience, New York (1962).
17
6.Saunders J.H. and Frish K.C. Polyurethanes. Part I. Chemistry, Interscience Publ., New York–London (1962).
7.Fedors R. “Effect of filler on the mechanical behavior of elastomers. Relationships between the small strain modulus and the type and concentration of filler,” Polymer, 20, No. 3, 324-328 (1979).
8.Lewis T.B. and Nielsen L.E. “Viscosity of dispersions and aggregates of suspensions of spheres,” Trans. Soc. Rheology, 12, No. 3, 421-443 (1968).
9.Sato Y. and Furukawa J. “A molecular theory of filler reinforcement based upon the conception of internal deformation,” Rub. Chem. Technol., 36, No. 4, 1081-1106 (1963).
10.Fedors R. and Landel R. “Mechanical behavior of SBR-glass bead composites,” J. Polym. Sci., No. 3, 579-601 (1975).
11.Farris R.J. “The character of the stress–strain function for highly filled elastomers,” Trans. Soc. Rheology, 12, No. 2, 303-314 (1968).
12.Farris R.J. “The influence of vacuole formation on the response and failure of filled elastomers,” Trans. Soc. Rheology, 12, No. 2, 315-334 (1968).
13.Smith T.L. and Chy W.H. “Ultimate tensile properties of elastomers” J. Polym. Sci., Pt. A-2, 10, No. 1, 133-150 (1972).
14.Zabrodin V.B., Zykov V.I., and Chui G.N. “Molecular structure of cross-linked polymers,” Vysokomol. Soed., 17A, No. 1, 163-169 (1975).
Механика композитных материалов. 2012,T. 48, №. 3.
МЕХАНИЧЕСКИЕ СВОЙСТВА ЭЛАСТОМЕРА, НАПОЛНЕННОГО ТВЁРДЫМИ ЧАСТИЦАМИ
А. С. Ермилов, Э. М. Нуруллаев
Пермский государственный технический университет, Пермь, Россия
Получено физико-механическое описание механических характеристик трёхмерно сшитого пластифицированного эластомера, наполненного твёрдыми частицами. Учтены основные структурные параметры композиции и температурно-скоростная эквивалентность в условиях одноос-
18
ного растяжения образца. Даны примеры решения прямой и обратной задач прогнозирования.
Ключевые слова: наполненный эластомер, механика, влияние структуры.
ВВЕДЕНИЕ
Полимерные композиционные материалы на основе эластомерной матрицы, наполненной твёрдыми частицами, находят широкое применение в деталях и узлах различных конструкций автомобилестроения, авиастроения, судостроения. Абразивные композиции, содержащие корунд (оксид алюминия), позволяют осуществлять высококачественное шлифование в машиностроении. Эластомеры, наполненные диоксидом кремния различных видов и фракционного состава, используются в строительной индустрии, включаяпокрытиезданийинастилвспортивных сооружениях.
Механические характеристики указанных материалов существенно влияют на эксплуатационный ресурс конкретной детали или узла конструкции. При этом наиболее важными структурными факторами являются: молекулярное строение полимерной основы, вид и степень её пластификации, объёмная степень эффективного наполнения, зависящая от формы и фракционного состава частиц дисперсного наполнителя, а также физикохимического взаимодействия на границе «эластомер–наполнитель».
Феноменологическое уравнение Муни–Ривлина, приведённое в обзоре Трелоара [1], основанное на упругом потенциале деформированной межузловой цепи в полимерной сетке, формально удовлетворительно описывает связь между условным напряжением (силой растяжения, отнесённой к начальному сечению) и удлинением образца. Однако оно не отражает в явном виде влияния основных физико-химических параметров состава эластомерной композиции на указанную зависимость. В связи с этим целью работы явилась разработка физико-химических основ механического поведения эластомера, наполненного твёрдыми частицами, принимая в качестве функционального ядра уравнение Муни–Ривлина.
Для достижения указанной цели нами предложено:
– нормированная функция температурно-скоростной зависимости «физической» (межмолекулярной) составляющей поперечных связей
вполимерном связующем;
–аналитическое соотношение между условным напряжением и удлинением, учитывающее усиление пластифицированной эластомерной матрицы частицами твёрдых компонентов.
19
ФИЗИКО-МАТЕМАТИЧЕСКОЕ ОПИСАНИЕ
Рассмотрим структурно-механическое поведение трёхмерно сшитого пластифицированного эластомера, наполненного твёрдыми частицами, в условиях одноосного растяжения. Упругий потенциал полимерной пространственной сетки, на основе которого Муни и Ривлин вывели своё уравнение, имеет вид:
U C1(I1 3) C2 (I2 3), |
(1) |
где U – упругий потенциал межузловой молекулярной цепи, равный внутренней энергии, накопленной за счёт работы, совершаемой при растяжении образца; I1, I2 – инварианты тензора удлинений второго ранга,
когда относительные удлинения (обозначим, xx 1, yy 2, zz 3) направлены параллельно осям декартовой системы координат; C1, C2 –
половины параметров, входящих в уравнение Муни-Ривлина. В свою очередь, указанные инварианты равны:
I1 12 22 32; I2 12 22 22 32 32 12, причём I3 12 22 32 1.
При этом относительные удлинения ( i ) связаны с относительными деформациями ( i ) следующим образом:
2 |
1 2 ; |
2 |
1 2 |
; |
2 |
1 2 |
3 |
1 |
1 |
2 |
2 |
|
3 |
|
при коэффициенте Пуассона, равном 0,5.
Соответствующие параметры (2C1 и 2C2) позволили Муни и Ривлину записать своё уравнение:
(2C 2C 1)( 2), |
(2) |
|
1 |
2 |
|
где σ – условное напряжение (сила растяжения, отнесённая к начальному сечению образца).
До сих пор параметры 2С1 и 2С2 связывались с химической и «физической» (межмолекулярной) составляющими поперечных связей в полимерной основе связующего.
Действительно, из формулы (2) формально следует выражение:
2C ( 2) 2C 1 |
( 2), |
(3) |
|
1 |
2 |
|
|
20