
Книги по МРТ КТ на английском языке / Functional Neuroimaging in Child Psychiatry Ernst 1 ed 2000
.pdf
264 B. S. Peterson and P. Thomas
Lim, S. H., Dinner, D. S., Pillay, P. K. et al., (1994). Functional anatomy of the human supplementary sensorimotor area: results of extraoperative electrical stimulation.
Electroencephalogr. Clin. Neurophysiol., 91, 179±93.
Lowe, T. L., Cohen, D. J., Detlor, J., Kremenitzer, M.W. and Shaywitz, B. A. (1982). Stimulant medications precipitate Tourette's syndrome. J. Am. Med. Assoc., 247, 1168±9.
Luria, A. R. (1980). Higher Cortical Functions in Man. New York: Basic Books.
Malison, R. T., McDougle, C. J., van Dyck, C. H. et al. (1995).
123IBeta-CIT SPECT imaging demonstrates increased striatal dopamine transporter binding in Tourette's syndrome. Am. J. Psychiatry, 152, 1359±61.
McLean, P. and Delgado, J. (1953). Electrical and chemical stimulation of frontotemporal portion of limbic system in the waking animal. Electroencephalogr. Clin. Neurophysiol., 5, 91±100.
Merzenich, M. M., Kaas, J. H., Wall, J. T., Sur, M., Nelson, R. J. and Felleman, D. J. (1983). Progression of change following median nerve section in the cortical representation of the hand in areas 3b and 1 in adult owl and squirrel monkeys. Neuroscience, 10, 639±65.
Mishkin, M. and Manning, F. (1978). Nonspatial memory after selective prefrontal lesions in monkeys. Brain Res., 143, 313±23.
Moran, J. and Desimone, R. (1985). Selective attention gates visual processing in the extrastriate cortex. Science, 229, 782±4.
Moriarty, J., Campos Costa, D., Schmitz, B., Trimble, M. R., Ell, P. J. and Robertson, M. M. (1995). Brain perfusion abnormalities in Gilles de la Tourette's syndrome. Br. J. Psychiatry, 167, 249±54.
Moriarty, J., Eapen, V., Costa, D. C. et al. (1997). HMPAO SPET does not distinguish obsessive-compulsive and tic syndromes in families multiply aVected with Gilles de la Tourette's syndrome.
Psychol. Med., 27, 737±40.
Nomoto, F. and Machiyama, Y. (1990). An epidemiological study of tics. Jap. J. Psychiatr. Neurol., 44, 649±55.
Obeso, J. A., Rothwell, J. C. and Marsden, C. D. (1981). Simple tics in Gilles de la Tourette's syndrome are not prefaced by a normal premovement EEG potential. J. Neurol. Neurosurg. Psychiatry,
44, 735±8.
Pardo, J. V., Pardo, P. J., Janer, K. W. and Raichle, M. E. (1990). The anterior cingulate cortex mediates processing selection in the Stroop attentional conXict paradigm. Proc. Natl. Acad. Sci. USA,
87, 256±9.
Parent, A. and Hazrati, L. (1995a). Functional anatomy of the basal ganglia. I. The cortico-basal ganglia-thalamo-cortical loop.
Brain Res. Rev., 20, 91±127.
Parent, A. and Hazrati, L.-N. (1995b). Functional anatomy of the basal ganglia: II. The place of subthalamic nucleus and external pallidum in basal ganglia circuitry. Brain Res. Rev., 20, 128±54.
Park, S., Como, P. G., Cui, L. and Kurlan, R. (1993). The early course of the Tourette's syndrome clinical spectrum. Neurology, 43, 1712±15.
Pauls, D. L., Towbin, K. E., Leckman, J. F., Zahner, G. E. and Cohen, D. J. (1986). Gilles de la Tourette's syndrome and obsessive-com-
pulsive disorder. Evidence supporting a genetic relationship.
Arch. Gen. Psychiatry, 43, 1180±2.
Pauls, D. L., Raymond, C. L., Stevenson, J. M. and Leckman, J. F. (1991). A family study of Gilles de la Tourette syndrome. Am. J. Hum. Genet., 48, 154±63.
Pauls, D. L., Leckman, J. F. and Cohen, D. J. (1993). Familial relationship between Gilles de la Tourette's syndrome, attention deWcit disorder, learning disabilities, speech disorders, and stuttering.
J. Am. Acad. Child Adolesc. Psychiatry, 32, 1044±50.
Petersen, S. E., Fox, P. T., Snyder, A. Z. and Raichle, M. E. (1990). Activation of extrastriate and frontal cortical areas by visual words and word-like stimuli. Science, 249, 1041±4.
Peterson, B. S., Leckman, J. F., Scahill, L. et al. (1992). Steroid hormones and CNS sexual dimorphisms modulate symptom expression in Tourette's syndrome. Psychoneuroendocrinology,
17, 553±63.
Peterson, B., Riddle, M. A., Cohen, D. J. et al. (1993). Reduced basal ganglia volumes in Tourette's syndrome using three-dimen- sional reconstruction techniques from magnetic resonance images. Neurology, 43, 941±9.
Peterson, B. S., Gore, J. C., Riddle, M. A., Cohen, D. J. and Leckman, J. F. (1994). Abnormal magnetic resonance imaging T2 relaxation time asymmetries in Tourette's syndrome. Psychiatr. Res. Neuroimaging, 55, 205±21.
Peterson, B. S., Bronen, R. A. and Duncan, C. C. (1996). Three cases of Gilles de la Tourette's syndrome and obsessive-compulsive disorder symptom change associated with paediatric cerebral malignancies. J. Neurol. Neurosurg. Psychiatry, 61, 497±505.
Peterson, B. S., Skudlarski, P., Anderson, A. W. et al. (1998). A functional magnetic resonance imaging study of tic suppression in Tourette syndrome. Arch. Gen. Psychiatry, 55, 326±33.
Peterson, B. S., Skudlarski, P., Zhang, H., Gatenby, J. C., Anderson, A. W. and Gore, J. C. (1999). An fMRI study of Stroop word-color interference: evidence for cingulate subregions subserving multiple distributed attentional systems. [Priority Communication] Biol. Psychiatry, 45, 1237±58.
Price, R. A., Kidd, K. K., Cohen, D. J., Pauls, D. L. and Leckman, J. F. (1985). A twin study of Tourette syndrome. Arch. Gen. Psychiatry,
42, 815±20.
Rauch, S. L., Jenike, M. A., Alpert, N. M. et al. (1994). Regional cerebral blood Xow measured during symptom provocation in obsessive-compulsive disorder using oxygen 15-labeled carbon dioxide and positron emission tomography. Arch. Gen. Psychiatry, 51, 62±70.
Rauch, S. L., Baer, L., Cosgrove, G. R. and Jenike, M. A. (1995). Neurosurgical treatment of Tourette's syndrome: a critical review. Compr. Psychiatry, 36, 141±56.
Richardson, E. P. (1982). Neuropathological studies of Tourette syndrome. In Gilles de la Tourette Syndrome, eds. A. J. FriedhoV and T. N. Chase, pp. 83±7. New York: Raven.
Riddle, M. A., Anderson, G. M., McIntosh, S., Harcherik, D. F., Shaywitz, B. A. and Cohen, D. J. (1986). Cerebrospinal Xuid monoamine precursor and metabolite levels in children treated for leukemia: age and sex eVects and individual variability. Biol. Psychiatry, 21, 69±83.
Tourette's syndrome |
265 |
|
|
Riddle, M. A., Rasmusson, A. M., Woods, S. W. and HoVer, P. B. (1992). SPECT imaging of cerebral blood Xow in Tourette syndrome. Adv. Neurol., 58, 207±11.
Robertson, M., Doran, M., Trimble, M. and Lees, A. J. (1990). The treatment of Gilles de la Tourette syndrome by limbic leucotomy.
J. Neurol. Neurosurg. Psychiatry, 53, 691±4.
Rosvold, H. and Mishkin, M. (1961). Nonsensory eVects of frontal lesions on discrimination learning and performance. In Brain Mechanisms and Learning, ed. J. Delafresnaye. Oxford: Blackwell.
Rubin, R. T., Ananth, J., Villanueva-Meyer, J., Trajmar, P. G. and Mena, I. (1995). Regional [133]Xenon cerebral blood Xow and cerebral [99m]Tc-HMPAO uptake in patients with obsessivecompulsive disorder before and during treatment. Biol. Psychiatry, 38, 429±37.
Sawle, G. V., Lees, A. J., Hymas, N. F., Brooks, D. J. and Frackowiak, R. S. (1993). The metabolic eVects of limbic leucotomy in Gilles de la Tourette syndrome. J. Neurol. Neurosurg. Psychiatry, 56, 1016±19.
Schwartz, J. M., Stoessel, P. W., Baxter, L. R., Martin, K. M. and Phelps, M. E. (1996). Systematic changes in cerebral glucose metabolic rate after successful behavior modiWcation treatment of obsessive-compulsive disorder. Arch. Gen. Psychiatry, 53, 109±13.
Seeman, P., Bzowej, N. H., Guan, H. C. et al. (1987). Human brain dopamine receptors in children and aging adults. Synapse, 1, 399±404.
Shaywitz, B. A., Cohen, D. J., Leckman, J. F.,Young, J. G. and Bowers, M., Jr (1980). Ontogeny of dopamine and serotonin metabolites in the cerebrospinal Xuid of children with neurological disorders. Dev. Med. Child Neurol., 22, 748±54.
Shulman, G. L., Corbetta, M., Buckner, R. L. et al. (1997a). Topdown modulation of early sensory cortex. Cereb. Cortex, 7, 193±206.
Shulman, G. L., Fiez, J. A., Corbetta, M. et al. (1997b). Common blood Xow changes across visual tasks: II. Decreases in cerebral cortex. J. Cogn. Neurosci., 9, 648±63.
Sieg, K. G., Buckingham, D., GaVney, G. R., Preston, D. F. and Sieg, K. G. (1992). Tc-99m HMPAO SPECT brain imaging of Gilles de la Tourette's syndrome. Clin. Nucl. Med., 18, 255.
Singer, H. S., Hahn, I. H. and Moran, T. H. (1991). Abnormal dopamine uptake sites in postmortem striatum from patients with Tourette's syndrome. Ann. Neurol., 30, 558±62.
Singer, H. S., Wong, D. F., Brown, J. E. et al. (1992). Positron emis-
sion tomography evaluation of dopamine D-2 receptors in adults with Tourette syndrome. Adv. Neurol., 58, 233±9.
Singer H. S., Reiss, A. L., Brown, J. E. et al. (1993). Volumetric MRI changes in basal ganglia of children with Tourette's syndrome.
Neurology, 43, 950±6.
Smith, S. J. M. and Lees, A. J. (1989). Abnormalities of the blink reXex in Gilles de la Tourette syndrome. J. Neurol. Neurosurg. Psychiatry, 52, 895±8.
Spitzer, H., Desimone, R. and Moran, J. (1988). Increased attention enhances both behavioral and neuronal performance. Science,
240, 338±40.
Stoetter, B., Braun, A. R., Randolph, C. et al. (1992). Functional neuroanatomy of Tourette syndrome. Limbic-motor interactions studied with FDG PET. Adv. Neurol., 58, 213±26.
Swedo, S. E., Schapiro, M. B., Grady, C. L. et al. (1989). Cerebral glucose metabolism in childhood-onset obsessive-compulsive disorder. Arch. Gen. Psychiatry, 46, 518±23.
Swedo, S. E., Pietrini, P., Leonard, H. L. et al. (1992). Cerebral glucose metabolism in childhood-onset obsessive-compulsive disorder. Revisualization during pharmacotherapy. Arch. Gen. Psychiatry, 49, 690±4.
Sweet, R. D., Bruun, R., Shapiro, E. and Shapiro, A. K. (1976). Presynaptic catecholamine antagonists as treatment for Tourette syndrome: eVects of alpha-methyl-para-tyrosine and tetrabenazine. Arch. Gen. Psychiatry, 31, 857±61.
Talairach, J., Bancaud, J., Geier, S. et al. (1973). The cingulate gyrus and human behavior. Electroenceph. Clin. Neurophys., 34, 45±52.
Talbot, J. D., Marrett, S., Evans, A. C., Meyer, E., Bushnell, M. C. and Duncan, G. H. (1991). Multiple representations of pain in human cerebral cortex. Science, 251, 1355±8.
Tasker, R. R. and Dostrovsky, J. O. (1993).What goes on in the motor thalamus? Stereotact. Funct. Neurosurg., 60, 121±6.
Turjanski, N., Sawle, G. V., Playford, E. D. et al. (1994). PET studies of the presynaptic and postsynaptic dopaminergic system in Tourette's syndrome. J. Neurol. Neurosurg. Psychiatry, 57, 688±92.
Vogt, B. A., Finch, D. M. and Olson, C. R. (1992). Functional heterogeneity in cingulate cortex: the anterior executive and posterior evaluative regions. Cereb. Cortex, 2, 435±43.
Wolf, S. S., Jones, D. W., Knable, M. B. et al. (1996). Tourette syndrome: prediction of phenotypic variation in monozygotic twins by caudate nucleus D2 receptor binding. Science, 273, 1225±7.
Wong, D. F., Singer, H. S., Brandt, J. et al. (1997). D2-like dopamine receptor density in Tourette syndrome measured by PET. J. Nucl. Med., 38, 1243±7.
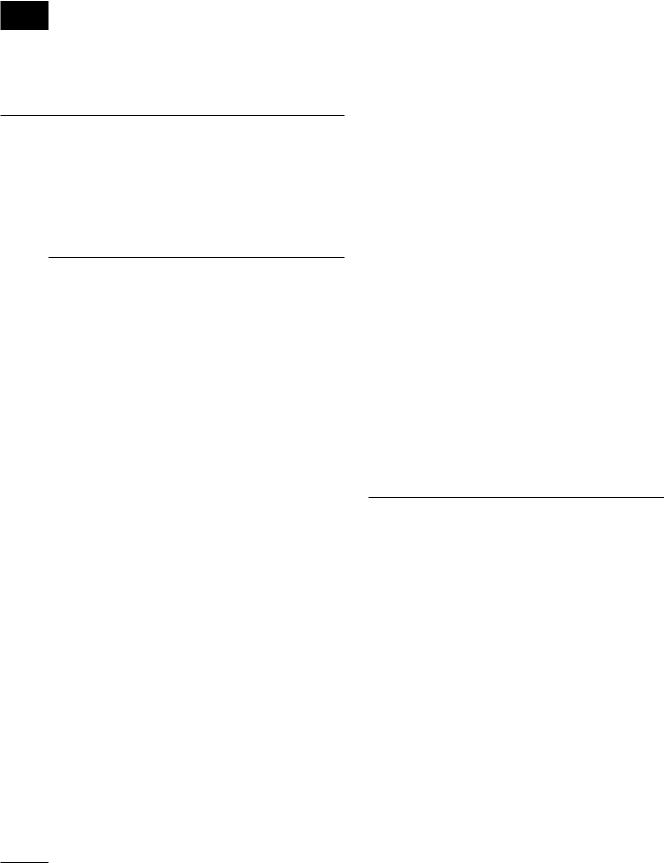
15
Dyslexia: conceptual issues and psychiatric comorbidity
Frank B. Wood and D. Lynn Flowers
Introduction
Until the advent of technology for direct imaging of localized anatomically speciWc brain function became available in the 1970s, the clinico-pathologic correlation method of Charcot and his contemporaries, whereby CNS lesions on autopsy were correlated with behavioral deWcits in life, formed the gold standard of clinical neurology. To this day, if a neurologist claims that a patient's behavioral deWcit arises from a focal lesion, and if there is dispute over that claim, only an autopsy can settle the issue with satisfactory certainty. Structural imaging by computed tomography (CT) and especially by magnetic resonance imaging (MRI), of course, has become a widely acceptable substitute for the autopsy. It is nonetheless also widely accepted (and vigorously asserted whenever a lesion that is proposed to explain a patient's deWcit is not visualized on MRI) that some ªtrueº lesions can be invisible by MRI and hence will only ªshow upº on autopsy. Against this, technological advances mean that demonstration of a structural lesion by imaging or at autopsy may no longer be essential, as it is possible to show dysfunction of a brain region(s). A lesion might certainly still be implied, but the range of possible lesions could be much broader, to include subtler derangements of functional anatomy on levels of scale as small as the synapse itself, where the abnormality would only express itself when large aggregates of these synapses, or their associated neurons, functioned deviantly (thus making themselves visible to functional imaging). Similarly, abnormalities of neurotransmitter function would fall within this enlarged class of ªlesionº since they do represent ultrastructural variations at the cellular and synaptic level. These and similar abnormalities might never be visible on autopsy, not simply because of the microscopic scale but because the abnormality itself might only be observable in living tissue.
Psychiatric disorders ± a substantial subset of which have deWed consensual clinicopathologic correlational analysis on autopsy ± would be expected to yield their functional neuroanatomic secrets to investigation by the new technology. The basic changes in the brain might now be objectively demonstrated. To do this, clinico pathologic correlates between disordered behavior and altered functional imaging landscapes would need to be drawn, a process involving a number of diYculties: individual diVerences in cellular or synaptic physiology are incompletely understood, the relationships of such physiological events to overt behavior are not fully known, and the necessary mathematical descriptions are complex at best.
Models of cognitive deWcits
The range of neurobehavioral disorders that have been investigated by this technology has reXected two rather diVerent theoretical perspectives, seldom reconciled. The diVerence between these two perspectives can be highlighted by asking: what could happen to the brain that would create the appearance of selective cognitive deWcit, as in selective reading disability? There are two distinctly diVerent possibilities described by a modular cognitive model and an anatomic model.
The modular cognitive model
The modular cognitive model postulates that a circumscribed, relatively speciWc cognitive process is disrupted in relative isolation from other processes. To use Pennington's (1997) analogy, a Swiss Army knife breaks one of its blades. While there are plausible neurobehavioral examples (e.g., weakness in the left leg arising from a high cortical motor strip lesion), this notion is ordinarily considered crude and
266

Dyslexia 267
oversimpliWed when applied to more complex cognitive and behavioral processes. The modestly more elaborate version of this concept, however, is more acceptable; it stresses the disruption of some particular process or system that is involved in several behaviors of a general cognitive class, all of which are to some extent aVected. It seems fair to say that the present state in dyslexia research tends to adopt this view. It expects that one or a few relatively circumscribed cognitive impairments selectively impede the learning and execution of a range of reading and reading-related skills and that the ªdisorderº in dyslexia is limited to those impairments. Whether phonological, visual, or other processes are presumed to underlie dyslexia, the theories put forward all share this common modular cognitive assumption ± that dyslexia reXects the selective disruption of a cognitively identiWable, appropriately delimited system. (Note that this does not necessarily imply explicit subtyping, only diVerent mechanisms any one or several of which would aVect a given dyslexic individual.) The major current multiple mechanism theory, Wolf's (Wolf and Obregon, 1992) two-factor model, does stress separate phonological and rapid naming (Xuency) components, which may operate jointly or separately in particular individuals. Such multiple mechanism models do allow for more heterogeneity of deWcits among dyslexics, but in the larger scheme of things they still imply, at most, a relatively few circumscribed underlying deWcits that are cognitively deWned.
Genetic investigations of dyslexia have followed a similar pattern toward diVerentiation of underlying mechanism. Initially, the linkages to a putative gene that conferred risk for reading disability were pursued with relatively global reading phenotypes that used a combination of tests to deWne generalized impairment in reading. This approach was fruitful in establishing linkages involving general reading ability on chromosome 15 (Smith et al., 1983) and chromosome 6 (Cardon et al., 1994). Heterogeneity, however, was strongest in our report (Grigorenko et al., 1997) in which the linkage on chromosome 6 was most strong if the phenotype was phonemic awareness (deWned by tests such as isolating a single phoneme within a word), less strong but still signiWcant if the phenotype was phonologic decoding (deWned as nonword reading), and only barely signiWcant if the phenotype was deWned as single word reading. Conversely, the linkage on chromosome 15 involved a phenotype that could only be deWned by single word reading skill, not involving phonemic awareness or phonologic decoding at all. Subsequently, we have demonstrated yet another strong linkage on chromosome 1 involving a putative gene best characterized by a phenotype of phonologic decod-
ing, operationally deWned as nonword reading (E. L. Grigorenko et al., unpublished data). Such Wndings naturally suggest heterogeneity of underlying mechanisms, but the data also suggest overlaps or interactions among these mechanisms.
In general, whether the underlying mechanisms are one or many, and regardless of whether they are molecular (genetic) or molar (anatomic), the modular cognitive model views them as behaviorally speciWc. The predictions from this model are, therefore, inherently speciWc: cognitive competencies will have a one-to-one mapping to biological mechanisms.
The anatomic model
The anatomic model postulates that a deWnable anatomic territory in the brain suVers damage, disease, or other compromise of its structure or function, either mild or severe, and all behavioral processes depending on tissue in that territory are to some extent aVected. A simple example of this concept is a stroke. Vascular territories do not follow functional maps, so a stroke ± especially a large one ± impairs a diverse collection of processes that are uniWed only by their separate dependence on the aVected tissue. In milder lesions, it is often the case that one of the symptoms is more obvious, and that is what brings the patient to clinical attention. A mild left frontal stroke, for example, may induce halting, dysXuent speech as the main symptom, but an observant clinician would readily detect the mild but deWnite right upper extremity weakness as well and might also detect the depression that often arises in the aftermath of such lesions (Robinson and Price, 1982). The underlying mechanism of dysXuent speech might be sought and carefully characterized as to behavioral subtypes and critical loci of lesion, and this would be instructive for cognitive neuroscience. Nonetheless, no explanation of the mechanism of the speech disorder, however sophisticated, could explain the hemiparesis or even the depression, which is independent of the severity or even the presence of associated motor, language, or cognitive deWcit. At another level of analysis, the underlying mechanism is the stroke itself, and science also does well to search for such mechanisms. If applied to the case of dyslexia, this model would stress that the lesion causing dyslexia is likely also to be causing other comorbid deWcits.
As in the cognitive modular model above, there are also more complex and subtle versions of the anatomic model. One familiar version of this model in dyslexia research is the Galaburda et al. (1985) tradition stressing the diVuse changes in neuronal connectivity that can arise from circumscribed cortical ectopias of prenatal origin in
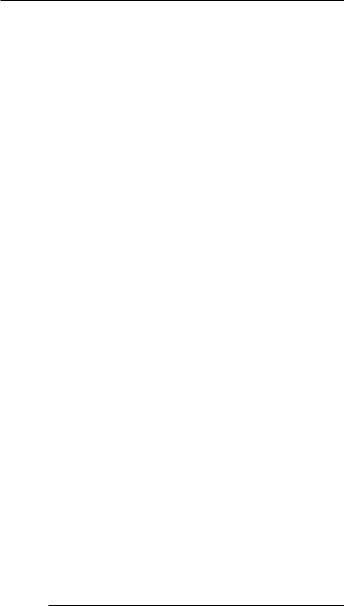
268F. B. Wood and D. L. Flowers
humans: inherent in this view has always been the proviso that the resultant cognitive deWcits would be diverse, unpredictable, and likely to extend well beyond any particular cognitive process. Another version of a subtle anatomic model would be a lesion in a speciWc neurotransmitter projection system that has diVuse consequences throughout the brain for a wide range of its functional capabilities. The degree to which an anatomic disruption is local, regional, or diVuse is seldom predictable a priori: location of lesion, therefore, becomes the fundamental empirical question that is a prerequisite to understanding the disease mechanism itself, as well as its behavioral consequences. Here, too, the symptom that comes to clinical attention may not be the only one that is present or important to the patient's life. Indeed, such additional unnoticed symptoms may be of particular importance precisely because they have impact on the patient's life without the patient's awareness. If there are comorbid deWcits, perhaps in the psychiatric domain, that accompany dyslexia, then the anatomic model assumes that it may not necessarily be possible to understand comorbidity to be arising from a common underlying ªprocessingº disorder. Instead, it may well be the case ± as in the above example of poststroke depression ± that the reading deWcit and the psychiatric comorbidity are independent expressions of the same general anatomic locus of dysfunction.
In contrast to the modular cognitive model then, the anatomic model predicts that the mappings from biology to behavior are always one to many, i.e., a given biological ªlesionº always results in several diVerent types and levels of behavioral proWles.
Psychiatric comorbidity
Central to a consideration of reading disability is the prospect of a diVerent perspective on the issue of comorbidity. Comorbidity is often considered in psychiatric nosology as a problem that threatens the accuracy and speciWcity of diagnosis. However, the anatomic model reviewed above suggests at least that the goal of speciWcity needs to be balanced with a goal of comprehensiveness. From our own work, we can formulate the following general suggestions for achieving that balance both in clinical and research situations. We will use two speciWc subtypes of dyslexia to illustrate that diVerential brain dysfunctions in speciWc subtypes of dyslexia have diVerential primary psychiatric consequences as well.
The subtypes or phenotypes in this illustration are (i) a primary phonemic awareness deWcit involving the inabil-
ity to segment spoken words orally into their constituent parts and (ii) a decoding deWcit involving the inability to pronounce written nonwords, despite intact phonemic awareness. (Grigorenko et al. (1997) gives a full description of the tests operationalizing these two phenotypes.) For the purposes of this analysis, the overlap between these two subtypes must be resolved since a phonemic awareness deWcit is itself one cause of decoding deWcit. Here anyone with a phonemic awareness de®cit is classiWed as aVected with the Wrst phenotype, regardless of whether they have a decoding deWcit. The second phenotype is reserved for those with a decoding deWcit without a phonemic awareness deWcit. Figures 15.1 and 15.2 show our present knowledge of the loci of anatomic hypometabolism in the two phenotypes.
The anatomic hypometabolism proWles could not diVer more: the phoneme awareness deWcit phenotype is associated with left posterior hypometabolism while the phonologic decoding deWcit phenotype is associated with right frontal hypometabolism. The heterogeneity is unambiguous, without overlap.
We next must ask whether other diVerential, anatomically plausible behavioral de®cits are also found. We ®nd that the phonemic awareness de®cit phenotype is often accompanied by symptoms of attention-deWcit hyperactivity disorder (ADHD), especially symptoms of the inattentive subtype, whereas the phonological decoding deWcit phenotype is accompanied only by some self-reported social withdrawal and shyness. We also Wnd the patients with phonemic awareness tend to have a variety of other perceptual ineYciencies, including visual ones, whereas those with decoding problems may be somewhat more motorically clumsy. Further testing is obviously required to conWrm the reliability and validity of these behavioral±anatomic associations, including their generalizability to other activating tasks or conditions of rest. (Note that if both types of dyslexic subject are combined into a single group, and this group is compared with normal control subjects, then the picture is simply a composite of the two regional syndromes and the simple contrast is statistically weakened because of the heterogeneity within dyslexia.)
The illustration permits the following broader suggestions:
1When children have deWcits involving the perception or isolation of phonemes (the children who cannot, for example, say what remains of a word after removing the initial consonant sound), it is as though they lack the elementary building blocks of reading. They are also likely to be unaware of other types of detail in the environment and may simulate or actually have a comorbid deWcit of ADHD, inattentive subtype. More broadly still, the inat-

Dyslexia 269
Fig. 15.1. Statistical parametric maps for the phonemic awareness subgroup showing regions of reduced glucose utilization during letter identiWcation, as measured with PET, in adults with a history of dyslexia and current deWcits in phonemic awareness (n5 16) relative to unaVected controls (n5 30). The regions shown reXect foci of at least 27 spatially contiguous voxels having a p#0.001 pixelwise group diVerence in metabolic rate following normalization for whole brain metabolism.
tentive subtype should be considered as a major risk whenever a learning disability involves inadequate perception.
2When children have a particular and relatively isolated deWcit in decoding without a phonemic awareness deWcit (the children who can decode the individual sounds but have diYculty blending them), it is as though they have a gestalt formation problem. They may be particularly prone to show this same problem in social relationships, seeming shy or clumsy. More generally, when children cannot integrate the components of written language, they may be at risk for a broad variety of coping deWcits. These are sometimes termed executive function deWcits, but that term may be too broad. In the present case at least, it looks more particularly like a gestalt formation problem or at least an organizational weakness. Still, such a deWcit alone can carry a major adaptive burden of inadequate coping, the symptoms of
which would extend far beyond reading.
In the light of this example, we will now review current results and the prospects for future progress. This essay
argues caution: although there has been important progress already, and some results could even be considered deWnitive, nevertheless there are still serious hazards to progress.
Progress and problems
The evidence for a genetic, and presumed neuroanatomic, basis for dyslexia is strong. There is abundant evidence to support a familial form of dyslexia (e.g., Hallgren, 1950; DeFries and Decker, 1982; DeFries et al., 1987; WolV and Melngailis, 1994), and there is ongoing investigation into the diVerential transmission of underlying reading acquisition skills (e.g., Olson et al., 1989; DeFries et al., 1991; Hohnen and Stevenson, 1995). Genetic linkage has further been demonstrated for global dyslexia phenotypes using markers on chromosome 15 (Smith et al., 1983; Fulker et al., 1991) and chromosome 6 (Cardon et al., 1994). Recent work shows that the linkages may involve more speciWc reading-related cognitive

270 F. B. Wood and D. L. Flowers
Fig. 15.2. Statistical parametric map for the phonological decoding subgroup showing regions of reduced glucose utilization during letter identiWcation, as measured with PET, in adults with a history of dyslexia and current deWcits in phonological decoding (but normal phonemic awareness) (n5 8) relative to unaVected controls (n5 30). The regions shown reXect foci of at least 27 spatially contiguous voxels having a p#0.001 pixelwise group diVerence in metabolic rate following normalization for whole brain metabolism.
phenotypes: phonemic awareness describes the chromosome 6 linkage especially well, single word reading the chromosome 15 linkage (Grigorenko et al., 1997), and phonologic decoding the chromosome 1 linkage (E. L. Grigorenko et al., unpublished data).
The apparent genetic heterogeneity of dyslexia raises the strong possibility that there are heterogeneous neurofunctional bases for dyslexia as well. By the term ªneurofunctionalº we mean ªpertaining to mechanisms whereby speciWc brain processes yield speciWc behavioral resultsº. Given heterogeneity, then serious methodologic problems follow as a direct consequence. There are three distinct possibilities, which can be described as three paradigms.
1The strong heterogeneity paradigm. This simplest case assumes that diVerent genetic subtypes inXict diVerent lesions, however subtle, each having diVerent neurofunctional consequences and each capable in its own right of inducing reading impairment. Any two-group normal versus dyslexic subject imaging study could fail, therefore, because the dyslexic group is not appropriately subtyped and the underlying mechanisms are obscured in the group comparison. This paradigm
assumes that reading can be poor for any of several distinct reasons, any one of which is a suYcient condition. Further investigation of this paradigm requires genetically relevant subgrouping, and it predicts the neurofunctional correlates to be distinctive to that subgrouping.
2 The converging pathway paradigm. This next case assumes that there are diVerent genetic mechanisms, but that they are nevertheless expressed in the same Wnal common pathway toward the same neuroanatomic lesion. In this case, a common localized functional deWcit is found across diVerent genetic subtypes. All subtypes impair the same process, which by deWnition and inexorable logic must then be a process required for adequate reading. This paradigm claims to have disclosed a common neuroanatomic mechanism or pathway from brain to reading disorder, but its further pursuit requires genetic subtyping for elucidation of the multiple pathways from genes to a common neuroanatomic mechanism. In this model, therefore, diVerent genes could impair the same anatomic locus in the brain, with identical behavioral results.

Dyslexia 271
3The tautology paradigm. This last case assumes underlying genetic and neuroanatomic heterogeneity, just as in the strong heterogeneity paradigm. In spite of that granted assumption, however, a common neurofunctional deWcit may nonetheless be shown, regardless of genetic subtype. The deWcit is by deWnition unrelated to the underlying genetic or neuroanatomic mechanism and, therefore, must be related only to the deWning attribute (reading disability) of the dyslexic versus normal comparison. If a localized functional deWcit is shown during reading task activation, then the case is a pure tautology: ªpeople who read poorly activate their brain reading mechanisms during tasks in an abnormal fashion, compared with those who read wellº. (How could it be otherwise, if the brain is the pathway to behavior?) To be sure, if several regions (i.e., a network) activate during normal reading and dyslexic subjects activate some portions of this network normally and others not at all, then, at least the search for important sites is narrowed. For example, Rumsey et al. (1997b) report that dyslexic subjects activate many portions of the neural networks that subserve reading as much as do normal subjects (e.g., some visual cortex and left inferior frontal cortex) and activate other regions (e.g., insula) even more than normal subjects. The abnormally activated areas are, in such a case, more interesting than the normally activated areas, although it remains unclear whether the abnormality is cause or consequence.
Problems with the paradigms
As it stands, the tautology paradigm cannot disclose the mechanism of dyslexia. As an example, let us assume that a phonological analysis task such as rhyming judgment, when competently performed, activates the left posterior superior perisylvian region. By deWnition, this means that in normal subjects the phonologic task causes more activation than a control task such as musical similarity judgments or than a resting baseline condition. Let us assume also that dyslexic subjects are known to be deWcient on this task, consistent with the general notion that most dyslexic subjects have some kind of phonologic processing problems. If dyslexic subjects show less than normal activity of Wernicke's area during rhyming task performance, then their brain activation proWle resembles that of controls who are performing either a nonphonologic task or are in a resting baseline condition. That might be simply because the dyslexic subjects were voluntarily not doing the phonologic task at all (refutable only if the experimenter monitors task performance) or were doing it carelessly with little
motivation or ªsetº for the task (refutable only if motivation, strategy, and accuracy of performance were not only monitored but demonstrably fully equal between normals and dyslexics). For all we know in such a case, the observed brain activation deWcit in dyslexia could have been environmentally induced by years of poor reading experience. In any case, the brain activation Wnding in itself means no more than the fact that the dyslexic subjects did not select Wernicke's area for activation. It speciWcally does not prove that they could not do so, still less that they were somehow prevented from doing so by a lesion in Wernicke's area.
When the tautology paradigm is pursued further, the issue of task speciWcity looms. If no neurofunctional deWcit is shown except on speciWc reading activation tasks, then the tautology is severe: it means simply that poor readers do not activate to reading tasks. If a neurofunctional deWcit is also shown on other tasks or states (e.g., music activation), and if this deWcit is nonetheless still independent of genetic subtypes, then some interesting content can be added to the tautology, which then becomes: ªpoor readers, whatever their etiology, are also deWcient in other areas, so they not only fail to activate certain brain mechanisms during reading but also during other tasks (e.g. music activation)º. The more such tasks are shown to diVerentiate the groups, the less conWdence there is in the speciWcity of the group classiWcation. In turn, adding to the list of deWcits leads to an unenlightening statement to the eVect that reading disabled individuals are simply less able in many ways.While this expands the content of the tautology, it certainly does not escape it. Historians of psychology will recognize the formal similarity of the tautology paradigm to the faculty psychology paradigm of the eighteenth century (ªa person is logical or musical according to the relative strength of his or her logical or musical facultiesº) or the phrenology paradigm of the nineteenth century (ªa person is logical or musical according to the relative size of his or her logical or musical brain areaº).
Both the converging pathway and tautology paradigms present an additional severe diYculty: although they are conceptually distinct, the two paradigms are indistinguishable without additional supporting evidence. In the example just discussed, the Wnding of a speciWc neurofunctional diVerence between normal and dyslexic subjects, on a reading-related task, was in itself no more than a tautology whereby poor readers activate their brains diVerently when engaged in a reading-related task. The neuroanatomic reason, if any, for that diVerence in activation remains completely obscure, and other approaches are required to elucidate it. There is no escape (i.e., no evidence with which to invoke a converging pathway paradigm and reject the tautology paradigm)

272F. B. Wood and D. L. Flowers
unless evidence about speciWc pathways from diVerent genes to a common neuroanatomic deWcit can be adduced. One example of such evidence might be the demonstration that genetically deWned subgroups of poor readers not only share common neurofunctional deWcits but also have separate additional traits speciWc to their genetic subtype. Consequently, in the literature review that follows, not only are the above explanatory paradigms generally at issue, but they are particularly so where an otherwise interesting Wnding may be no more than a neurobehavioral tautology.
Studies of normal language and reading
Logically, interest Wrst focuses on studies involving normal language processing. These show numerous examples of nonreading disabled subjects with left temporal activation during the execution of language tasks: word meaning tasks (Vanderberghe et al., 1996), phoneme monitoring (Sergent et al., 1992; Demonet et al., 1994a), rhyme judgments (Shaywitz, et al., 1995), word repetition (Herholz et al., 1994; Karbe et al., 1995), and reading aloud (Small et al., 1996). Left inferior parietal regions are also implicated in normal phonetic processing (Petersen et al., 1989; Demonet et al., 1994a; Zatorre et al., 1996), word retrieval (Warburton et al., 1996), viewing words (Menard et al., 1996), and oral reading (Bookheimer et al., 1995). A century of neurobehavioral theory and evidence leads to this expectation, and the expectation is conWrmed. Even in normal subjects, however, the broader relationships of tasks to regions are far from simple. On the one hand, some of the same regions show deactivation to relevant verbal tasks (e.g., oral pronunciation or lexical decision, Rumsey et al., 1997a). On the other hand, a wide variety of other regions is also activated by language stimuli. Numerous left hemisphere regions, other than temporal, respond to language processing: viewing words (Menard et al., 1996), reading pseudowords (Frith et al., 1995), speech from memory (Tamas et al., 1993), rhyme judgment (Paulesu et al., 1993; Shaywitz et al., 1995; Zatorre et al., 1996), and phoneme monitoring (Demonet et al., 1992, 1994b) all activate Broca's and related frontal areas, which is not surprising. Somewhat more surprising is a consistent but less commonly advertised Wnding: left or bilateral lingual and neighboring extrastriate cortex is also activated by a variety of language-related tasks: by reading, pronouncing, naming, or making phonologic or orthographic judgments about words, nonwords, idiosyncratically spelled words, or objects (Price, 1977; Bookheimer et al., 1995; Frith et al., 1995; Kiyosawa et al. 1996; Rumsey et al., 1997a). It is espe-
cially interesting that the nonvisual task of monitoring of speech and nonspeech sounds for the occurrence of target phonemes (Demonet et al., 1994b) also activates the lingual gyri. Other nonlexical visual±verbal tasks such as face identiWcation and categorizing visual objects (Sergent et al., 1992) also activate this region.
Language tasks also activate right hemisphere regions, conWrming that the left hemisphere is not alone in the processing of language-related stimuli. There is right hemisphere or bilateral participation in tasks requiring word repetition (Herholz et al., 1994; Karbe et al., 1995), phoneme monitoring (Demonet et al., 1994a), exemplar generation (Shaywitz et al., 1995), speech from memory (Tamas et al., 1993), listening to distorted spoken words (Howard et al., 1992), and executive functions involving naming or reading, such as the Stroop test (Bench et al., 1993). Speaking or reading aloud also activates sensorimotor cortices and supplementary motor areas of both hemispheres (e.g., Herholz et al., 1994; Price et al., 1994; Warburton et al., 1996). Furthermore, tasks requiring nonlexical processing also activate some of the same left hemisphere regions as are activated by language tasks in normal subjects: attending to shape is found to activate the left inferior parietal lobule (Corbetta et al., 1991) and processing pictures for meaning activates the left posterior inferior temporal sulcus (Vanderberghe et al., 1996). Silent naming of pictures also activates basal temporal cortex and the parietal operculum (Bookheimer et al., 1995).
Generally then, diVerent types of language tasks may activate the same left hemisphere regions ± whether classic language areas or not ± and a language task may activate bilateral or isolated right hemisphere regions as well. Nonlanguage tasks may also activate left hemisphere regions, overlapping with those seen in language activation tasks. To be sure, some of the variance is certainly accounted for by stimulus parameters (Ingvar et al., 1993), some by registration technique (Watson et al., 1993; Grabowski et al., 1995), and some by the nearly universal use of subtraction techniques (Poeppel, 1996; but see Demonet et al., 1993).
Additionally, as has been suggested (Poeppel, 1996), some of the variance in Wndings could be explained by the fact that it may be impossible to avoid engaging the whole of the language apparatus when presenting subjects with lexical materials. See Petersen and Fiez (1993) for a comprehensive review of their important series on processes involved in single word reading in normal subjects, illustrating many of these and other complexities and Demonet et al. (1993) for a review of broader language processing studies in normal subjects using positron emission tomography (PET).

Dyslexia studies
To date, the published functional neuroimaging studies of dyslexia have studied adult subjects virtually exclusively. Functional imaging work on dyslexia begins conceptually with an early PET study (Lubs et al., 1988) that followed logically from the genetic studies of the early 1980s implicating a linkage on chromosome 15 involving familial dyslexia (Smith et al., 1983). Lubs et al. (1988) compared the brain glucose proWles of six familial dyslexic subjects as they read aloud with the proWles of unaVected subjects who either read aloud or viewed pictures. The dyslexic subjects had lower left perisylvian and higher occipital glucose metabolism when compared with the oral reading control group, dyslexic subjects having a proWle more similar to that of control subjects who viewed pictures, suggesting that familial dyslexic subjects process less of the lexical or phonetic characteristics and more of the visual or pictorial characteristics of words. The same group (Gross-Glenn et al., 1991), also using PET assessment of glucose, found greater right than left prefrontal activity in both familial dyslexic subjects and controls, less prefrontal asymmetry in the dyslexic group, and greater bilateral activity in the lingual gyri of the occipital lobe in the dyslexic subjects, especially pronounced on the left. This was interpreted as ineYcient, hence metabolically more demanding, visual word form processing within the primary visual to temporal lobe pathway and suggested a visual system impairment in dyslexia. Visual pathway abnormalities in dyslexia have also been reported by Eden et al. (1996). The regions of excess or deWcient activity in the left hemisphere in dyslexia in all these studies have extended beyond the left perisylvian region to include more posterior regions of cortex usually implicated in visual rather than auditory processing. (See Kinsbourne et al. (1991) for the analogous behavioral Wnding of considerable breadth of dyslexic deWcit, not at all limited to phonologic or even to verbal processes.) Nonetheless, perisylvian and related regions have certainly also been implicated. In another relatively early study from our own group (Flowers et al., 1991), subjects performed an auditory-orthographic task during the measurement of blood Xow using xenon inhalation, and the dyslexic subjects showed abnormal task-related activity in the left hemisphere. In that study, however, lower than normal left posterior superior temporal activation was accompanied by greater than normal activation in the left angular gyrus regions. Interestingly, there were also right hemisphere group distinctions in anterior and posterior perisylvian regions that were unrelated to task performance measures, with the posterior one being related to a measure of anxiety in the dyslexia sample. In a subsequent PET study using a
Dyslexia 273
phoneme discrimination task, we found another abnormality of excess: in the bilateral medial posterior temporal lobes of dyslexic subjects (Hagman et al., 1992). Measures of regional cerebral blood Xow (rCBF) using xenon in the same phoneme discrimination task in a larger number of subjects showed that, while there were no group mean diVerences in task performance or blood Xow values, left superior temporal lobe activation was positively correlated with task accuracy in dyslexic subjects but negatively correlated with task accuracy in controls: as though dyslexic subjects actually depended more on the left temporal language cortex than normal readers (Wood et al., 1991). (See Wood (1990) for a review of this and related interpretive issues including abnormalities of excess.)
Ingvar et al. (1995) also reported lower than normal activation in the middle temporal gyrus of dyslexic subjects during a diVerent task, that of reading either aloud or silently. Paulesu et al. (1996) reported that compensated dyslexic subjects did not show normal activation of the left insular area during either a rhyming task or a visual letter memory task; they also showed abnormal hypoactivation of the right hemisphere during a phonologic memory task. However, they did Wnd Broca's area activation during a rhyming task and temporoparietal activation during a letter memory task in dyslexic subjects. While nonreading disabled control subjects activated the anterior and posterior perisylvian areas simultaneously, the compensated dyslexic group did not. That fact and the lack of insular activity suggested to them that dyslexia is a ªdisconnection syndromeº, or at least a disorder in which activation is fragmented and not as uniWed as in normals. More recently, Shaywitz (1998) has reported a somewhat similar dissociation in dyslexia of anterior and posterior excess and deWcit, respectively, involving phonologic activation using fMRI subtraction.
In all the above studies, however, there is little hard evidence with which to refute a tautology paradigm or compel adoption of a converging pathway paradigm; the absence of explicit heterogeneity of brain activation proWles within dyslexia eliminates the strong heterogeneity paradigm. Although abnormalities of excess represent a degree of additional complexity in the interpretation of the underlying deWcits, they still are vulnerable to the circular interpretation that dyslexic individuals fail to activate left hemisphere regions because they are dyslexic (or dysphonologic, hypoverbal, etc.).
Rumsey and colleagues have made the major sustained, contribution to date in a series of studies in the investigation of dyslexia brain proWles. Their series of PET rCBF studies has demonstrated a speciWc temporoparietal deWcit in severely reading-disabled men in spite of partial