
- •Abstract
- •Acknowledgements
- •Highlights
- •Executive summary
- •Findings and recommendations
- •Electric mobility is developing at a rapid pace
- •Policies have major influences on the development of electric mobility
- •Technology advances are delivering substantial cost reductions for batteries
- •Strategic importance of the battery technology value chain is increasingly recognised
- •Other technology developments are contributing to cost cuts
- •Private sector response confirms escalating momentum for electric mobility
- •Outlooks indicate a rising tide of electric vehicles
- •Electric cars save more energy than they use
- •Electric mobility increases demand for raw materials
- •Managing change in the material supply chain
- •Safeguarding government revenue from transport taxation
- •New mobility modes have challenges and offer opportunities
- •References
- •Introduction
- •Electric Vehicles Initiative
- •EV 30@30 Campaign
- •Global EV Pilot City Programme
- •Scope, content and structure of the report
- •1. Status of electric mobility
- •Vehicle and charger deployment
- •Light-duty vehicles
- •Stock
- •Cars
- •Light-commercial vehicles
- •Sales and market share
- •Cars
- •Light-commercial vehicles
- •Charging infrastructure
- •Private chargers
- •Publicly accessible chargers
- •Small electric vehicles for urban transport
- •Stock and sales
- •Two/three-wheelers
- •Low-speed electric vehicles
- •Charging infrastructure
- •Buses
- •Stock and sales
- •Charging infrastructure
- •Trucks
- •Stock and sales
- •Charging infrastructure
- •Other modes
- •Shipping
- •Aviation
- •Energy use and well-to-wheel GHG emissions
- •Electricity demand and oil displacement
- •Well-to-wheel GHG emissions
- •References
- •2. Prospects for electric mobility development
- •Electric mobility targets: Recent developments
- •Country-level targets
- •City-level targets
- •Policy updates: Vehicles and charging infrastructure
- •Charging standards
- •Hardware
- •Communication protocols
- •Supporting policies
- •Canada
- •China
- •Vehicle policies
- •Charging infrastructure policies
- •Industrial policies
- •European Union
- •Vehicle policies
- •Charging infrastructure policies
- •Industrial policy
- •India
- •Vehicle policies
- •Charging infrastructure policies
- •Japan
- •Vehicle policies
- •Charging infrastructure policies
- •Industrial policy
- •Korea
- •Vehicle policies
- •Charging infrastructure
- •Industrial policy
- •United States
- •Vehicle policies
- •Charging infrastructure
- •Industrial policy
- •Other countries
- •The emergence of a Global Electric Mobility Programme
- •Industry roll-out plans
- •Vehicles
- •Light-duty vehicles
- •Two/three-wheelers
- •Buses
- •Trucks
- •Automotive batteries
- •Charging infrastructure
- •References
- •3. Outlook
- •Scenario definitions
- •Electric vehicle projections
- •Policy context for the New Policies Scenario
- •Global results
- •Two/three-wheelers
- •Light-duty vehicles
- •Buses
- •Trucks
- •Regional insights
- •China
- •Europe
- •India
- •Japan
- •United States and Canada
- •Other countries
- •Implications for automotive batteries
- •Capacity of automotive batteries
- •Material demand for automotive batteries
- •Charging infrastructure
- •Private chargers
- •Light-duty vehicles
- •Buses
- •Private charging infrastructure for LDVs and buses
- •Publicly accessible chargers for LDVs
- •Impacts of electric mobility on energy demand
- •Electricity demand from EVs
- •Structure of electricity demand for EVs in the New Policies Scenario
- •Structure of electricity demand for EVs in the EV30@30 Scenario
- •Implications of electric mobility for GHG emissions
- •References
- •4. Electric vehicle life-cycle GHG emissions
- •Context
- •Methodology
- •Key insights
- •Detailed assessment
- •Life-cycle GHG emissions: drivers and potential for emissions reduction
- •Effect of mileage on EV life-cycle GHG emissions
- •Effect of vehicle size and power on EV life-cycle emissions
- •Effect of power system and battery manufacturing emissions on EV life-cycle emissions
- •References
- •5. Challenges and solutions for EV deployment
- •Vehicle and battery costs
- •Challenge
- •EV purchase prices are not yet competitive with ICE vehicles
- •Indications from the total cost of ownership analysis
- •Effect of recent battery cost reductions on the cost gap
- •Impacts of developments in 2018 on the total cost of ownership
- •Solutions
- •Battery cost reductions
- •Reducing EV costs with simpler and innovative design architectures
- •Adapting battery sizes to travel needs
- •Supply and value chain sustainability of battery materials
- •Challenges
- •Solutions
- •Towards sustainable minerals sourcing via due diligence principles
- •Initiatives for better battery supply chain transparency and sustainable extractive activities
- •Bridging the gap between due diligence principles and on-the-ground actions
- •Battery end-of-life management
- •Implications of electric mobility for power systems
- •Challenges
- •Solutions
- •Potential for controlled EV charging to deliver grid services and participate in electricity markets
- •Enabling flexibility from EVs
- •Importance of policy actions to enable EV participation in markets
- •Government revenue from taxation
- •Challenges
- •Solutions
- •Near-term options
- •Long-term solutions
- •Shared and automated mobility
- •Challenges
- •Solutions
- •References
- •Statistical annex
- •Electric car stock
- •New electric car sales
- •Market share of electric cars
- •Electric light commercial vehicles (LCV)
- •Electric vehicle supply equipment stock
- •References
- •Acronyms, abbreviations and units of measure
- •Acronyms and abbreviations
- •Units of measure
- •Table of contents
- •List of Figures
- •List of Boxes
- •List of Tables
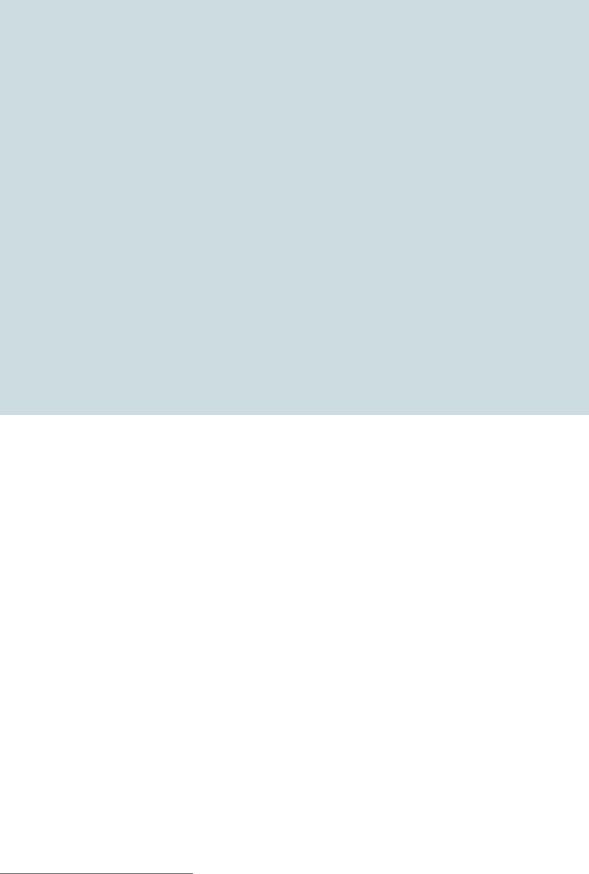
Global EV Outlook 2019 |
3. Outlook |
|
|
|
|
privately owned. Given the large energy requirements of both mediumand heavy-freight trucks (roughly 1 and 1.4 kWh/km, respectively), it is also likely that, similar to buses, trucks will mostly rely on fast chargers.
Electricity demand estimated for trucks by 2030 is about 13 TWh in the New Policies Scenario and 72 TWh in the EV30@30 Scenario. The daily energy demand requirements depend on the allelectric range of the vehicles and the characteristics of their batteries. If PHEVs trucks have allelectric daily mileages of 30 km for medium-freight trucks and 15 km for heavy-freight trucks, and BEVs have a range of 150 km, a usage frequency of five days a week and 50 weeks a year would be compatible with all-electric mileages of 7 500 and 3 750 km/year for medium and heavy PHEV trucks, and a maximum of 37 500 km for BEV trucks. With a 50 kW charger, PHEVs would need less than one hour to charge, and BEVs would need two to three hours. With increasing charging power capacity, these times would reduce, allowing more opportunities to share charging infrastructure.
Assuming caps of 10 PHEV trucks and 10 BEV medium trucks (with a 165 kW average power by 2030) per charger and five BEV heavy trucks to be sharing chargers with a 450 kW power rate, and taking into account of the energy demand from the electric truck uptake considered in the scenarios would lead to 140 000 chargers deployed in the New Policies Scenario and 350 000 in the EV30@30 Scenario, mostly for medium trucks. The power capacity coupled with these chargers in 2030 would reach 27 GW in the New Policies Scenario and 67 GW in the EV30@30 Scenario.
Publicly accessible chargers for LDVs
Publicly accessible charging points for LDVs are complementary to private chargers. They are important enablers of the use of BEVs over long distances and by consumers that are interested in EVs but do not have the possibility to rely on private (home or workplace) chargers.
The projections for publicly accessible LDV chargers in both the New Policies and the EV30@30 scenarios build on the following considerations:
•Most countries are assumed to have a ratio of publicly accessible chargers converging towards one charger per ten electric LDVs in 2030, i.e. the value indicated by the targets in China and the European Union.
•The share of fast chargers per EV is stable and equal to a value of 10% of the distance driven by EVs in all global regions except China and Japan, where the same share is assumed equal to 20%. The 10% value is consistent with the much higher frequency of use of home and
workplace chargers observed in the Nordic region (IEA, 2018c) and larger amounts of kWh per charge in the case of fast charging.24 The higher value for China and Japan is based on higher reliance on fast charging that is observed today.
•Publicly accessible slow chargers are level 2 chargers with an average power rate increasing from about 3.7 kW today to 7.4 kW in 2030.
•DC fast chargers are subject to a progressive upgrade to ultra-fast and high power chargers, from an average of 50 kW in 2018 to almost 100 kW by 2030. If the capacity utilisation of
24 The 10% value is also used in a recent study developed for the European Commission (Cambridge Econometrics, 2018).
PAGE | 138
IEA. All rights reserved.
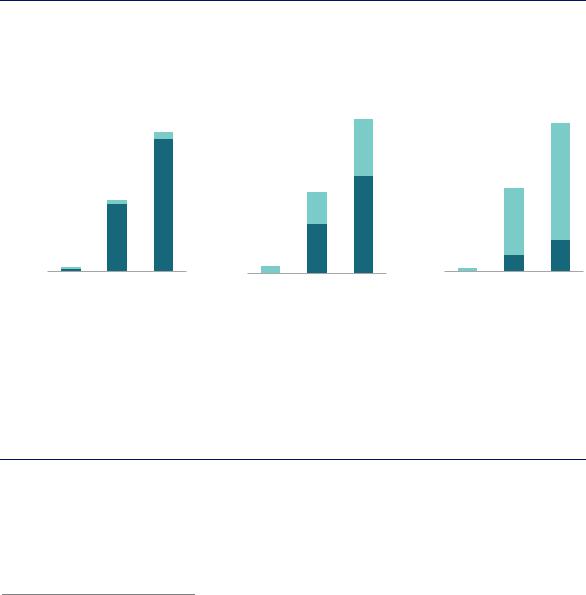
Global EV Outlook 2019 |
3. Outlook |
fast chargers remains unchanged, this leads to a significant reduction in the number of publicly accessible fast charging points. This also brings down the share of fast chargers in the total of all publicly accessible chargers.25
In the New Policies Scenario, the number of publicly accessible chargers for LDVs resulting from these considerations increases from about 560 000 today to 10 million in 2030 (Figure 3.7). Slow chargers account for about 95% of the total publicly accessible charging infrastructure in 2030, up from 70% today. The power capacity of LDV public charging infrastructure reaches 113 GW in 2030, with fast chargers accounting for almost 40% of the total. The total energy consumed by publicly accessible chargers for LDVs is 70 TWh in 2030, with fast chargers delivering about 80% of it.
Figure 3.7. Number of publicly accessible chargers for LDVs, relative power capacity and energy demand by scenario, 2018-30
|
|
|
Number of chargers |
|
|
|
Power capacity |
|
|
|
Energy demand |
|
|||||||||||||||
ofNumberchargers (millions) |
25 |
|
|
|
|
|
|
|
powerChargingcapacity (GW) |
250 |
|
|
|
|
|
|
|
|
Energysupplied (TWh) |
150 |
|
|
|
|
|
|
|
|
|
|
|
|
|
|
|
|
|
|
|
|
|
|
|
|
|
||||||||||
|
|
|
|
|
|
|
|
|
|
|
|
|
|
|
|
|
|
|
|||||||||
5 |
|
|
|
|
|
|
|
50 |
|
|
|
|
|
|
|
|
30 |
|
|
|
|
|
|
|
|||
|
20 |
|
|
|
|
|
|
|
|
200 |
|
|
|
|
|
|
|
|
|
120 |
|
|
|
|
|
|
|
|
15 |
|
|
|
|
|
|
|
|
150 |
|
|
|
|
|
|
|
|
|
90 |
|
|
|
|
|
|
|
|
10 |
|
|
|
|
|
|
|
|
100 |
|
|
|
|
|
|
|
|
|
60 |
|
|
|
|
|
|
|
|
|
|
|
|
|
|
|
|
|
|
|
|
|
|
|
|
|
|
|
|
|||||||
|
|
|
|
|
|
|
|
|
|
|
|
|
|
|
|
|
|
|
|
|
|
||||||
|
0 |
|
|
|
|
|
|
|
|
0 |
|
|
|
|
|
|
|
|
|
0 |
|
|
|
|
|
|
|
|
|
2018 |
|
2030 |
|
2030 |
|
|
|
|
|
|
|
|
|
|
|
|
2018 |
|
2030 |
|
2030 |
|
|||
|
|
|
|
|
|
|
2018 |
|
2030 |
|
2030 |
|
|
|
|
|
|
|
|||||||||
|
|
|
|
|
|
|
|
|
|
|
|
|
|
|
|
||||||||||||
|
|
|
|
|
|
|
|
|
|
|
|
|
|
|
|
|
|
|
|||||||||
|
|
|
|
|
NPS |
|
EV30@30 |
|
|
|
|
|
NPS |
|
EV30@30 |
|
|
|
|
|
NPS |
|
EV30@30 |
|
|||
|
|
|
|
|
|
|
|
|
Slow chargers |
|
|
|
|
|
|
Fast chargers |
|
|
|
|
|
||||||
|
|
|
|
|
|
|
|
|
|
|
|
|
|
|
|
|
|
|
|
||||||||
|
|
|
|
|
|
|
|
|
|
|
|
|
|
|
|
|
|
|
|
Notes: NPS = New Policies Scenario. The assumptions used for the development of these projections are included in the main text. Source: IEA analysis developed with the IEA Mobility Model (IEA, 2019a).
The number of public LDV chargers reaches 10 million in the New Policies Scenario and almost 20 million in the EV30@30 Scenario in 2030, of which most are slow chargers. The power capacity is more evenly distributed among the two types of publicly accessible chargers. Fast chargers serve the bulk of electricity demand.
In 2030, the number of publicly accessible LDV chargers is 7% of the total number of LDV chargers (public and private). The power capacity of publicly accessible LDV chargers accounts for 12% of the total and about 18% of the total electricity consumed by EVs.
25 With a constant share of electricity delivered through fast chargers, an increase in power rating for fast chargers translates into a reduction of the amount of publicly accessible fast chargers that serve the EV fleet, if compared with a situation where the increase in the average power rating of the fast chargers does not take place. With a 10% occupancy rate for a fast charger, a 10% share of the distance driven by one EV in Europe could be satisfied by either one 50 kW fast charger for 150 cars, one 175 kW fast charger for 500 cars or one 350 kW fast charger for 1 000 cars. Similar results are indicated in the analysis developed for the European Commission (Cambridge Econometrics, 2018).
PAGE | 139
IEA. All rights reserved.