
- •Abstract
- •Acknowledgements
- •Highlights
- •Executive summary
- •Findings and recommendations
- •Electric mobility is developing at a rapid pace
- •Policies have major influences on the development of electric mobility
- •Technology advances are delivering substantial cost reductions for batteries
- •Strategic importance of the battery technology value chain is increasingly recognised
- •Other technology developments are contributing to cost cuts
- •Private sector response confirms escalating momentum for electric mobility
- •Outlooks indicate a rising tide of electric vehicles
- •Electric cars save more energy than they use
- •Electric mobility increases demand for raw materials
- •Managing change in the material supply chain
- •Safeguarding government revenue from transport taxation
- •New mobility modes have challenges and offer opportunities
- •References
- •Introduction
- •Electric Vehicles Initiative
- •EV 30@30 Campaign
- •Global EV Pilot City Programme
- •Scope, content and structure of the report
- •1. Status of electric mobility
- •Vehicle and charger deployment
- •Light-duty vehicles
- •Stock
- •Cars
- •Light-commercial vehicles
- •Sales and market share
- •Cars
- •Light-commercial vehicles
- •Charging infrastructure
- •Private chargers
- •Publicly accessible chargers
- •Small electric vehicles for urban transport
- •Stock and sales
- •Two/three-wheelers
- •Low-speed electric vehicles
- •Charging infrastructure
- •Buses
- •Stock and sales
- •Charging infrastructure
- •Trucks
- •Stock and sales
- •Charging infrastructure
- •Other modes
- •Shipping
- •Aviation
- •Energy use and well-to-wheel GHG emissions
- •Electricity demand and oil displacement
- •Well-to-wheel GHG emissions
- •References
- •2. Prospects for electric mobility development
- •Electric mobility targets: Recent developments
- •Country-level targets
- •City-level targets
- •Policy updates: Vehicles and charging infrastructure
- •Charging standards
- •Hardware
- •Communication protocols
- •Supporting policies
- •Canada
- •China
- •Vehicle policies
- •Charging infrastructure policies
- •Industrial policies
- •European Union
- •Vehicle policies
- •Charging infrastructure policies
- •Industrial policy
- •India
- •Vehicle policies
- •Charging infrastructure policies
- •Japan
- •Vehicle policies
- •Charging infrastructure policies
- •Industrial policy
- •Korea
- •Vehicle policies
- •Charging infrastructure
- •Industrial policy
- •United States
- •Vehicle policies
- •Charging infrastructure
- •Industrial policy
- •Other countries
- •The emergence of a Global Electric Mobility Programme
- •Industry roll-out plans
- •Vehicles
- •Light-duty vehicles
- •Two/three-wheelers
- •Buses
- •Trucks
- •Automotive batteries
- •Charging infrastructure
- •References
- •3. Outlook
- •Scenario definitions
- •Electric vehicle projections
- •Policy context for the New Policies Scenario
- •Global results
- •Two/three-wheelers
- •Light-duty vehicles
- •Buses
- •Trucks
- •Regional insights
- •China
- •Europe
- •India
- •Japan
- •United States and Canada
- •Other countries
- •Implications for automotive batteries
- •Capacity of automotive batteries
- •Material demand for automotive batteries
- •Charging infrastructure
- •Private chargers
- •Light-duty vehicles
- •Buses
- •Private charging infrastructure for LDVs and buses
- •Publicly accessible chargers for LDVs
- •Impacts of electric mobility on energy demand
- •Electricity demand from EVs
- •Structure of electricity demand for EVs in the New Policies Scenario
- •Structure of electricity demand for EVs in the EV30@30 Scenario
- •Implications of electric mobility for GHG emissions
- •References
- •4. Electric vehicle life-cycle GHG emissions
- •Context
- •Methodology
- •Key insights
- •Detailed assessment
- •Life-cycle GHG emissions: drivers and potential for emissions reduction
- •Effect of mileage on EV life-cycle GHG emissions
- •Effect of vehicle size and power on EV life-cycle emissions
- •Effect of power system and battery manufacturing emissions on EV life-cycle emissions
- •References
- •5. Challenges and solutions for EV deployment
- •Vehicle and battery costs
- •Challenge
- •EV purchase prices are not yet competitive with ICE vehicles
- •Indications from the total cost of ownership analysis
- •Effect of recent battery cost reductions on the cost gap
- •Impacts of developments in 2018 on the total cost of ownership
- •Solutions
- •Battery cost reductions
- •Reducing EV costs with simpler and innovative design architectures
- •Adapting battery sizes to travel needs
- •Supply and value chain sustainability of battery materials
- •Challenges
- •Solutions
- •Towards sustainable minerals sourcing via due diligence principles
- •Initiatives for better battery supply chain transparency and sustainable extractive activities
- •Bridging the gap between due diligence principles and on-the-ground actions
- •Battery end-of-life management
- •Implications of electric mobility for power systems
- •Challenges
- •Solutions
- •Potential for controlled EV charging to deliver grid services and participate in electricity markets
- •Enabling flexibility from EVs
- •Importance of policy actions to enable EV participation in markets
- •Government revenue from taxation
- •Challenges
- •Solutions
- •Near-term options
- •Long-term solutions
- •Shared and automated mobility
- •Challenges
- •Solutions
- •References
- •Statistical annex
- •Electric car stock
- •New electric car sales
- •Market share of electric cars
- •Electric light commercial vehicles (LCV)
- •Electric vehicle supply equipment stock
- •References
- •Acronyms, abbreviations and units of measure
- •Acronyms and abbreviations
- •Units of measure
- •Table of contents
- •List of Figures
- •List of Boxes
- •List of Tables

Global EV Outlook 2019 |
1. Status of electric mobility |
exceed four seats. There are projects of hybrid 12-seater planes for short-haul flights, for instance from Zunum Aero, a start-up backed by Boeing (Reed, 2018).35 In principle, hybrid planes will be backed by a traditional kerosene engine for take-off and landing, and would rely entirely on electric propulsion in cruising mode. In the longer term, the start-up Wright Electric, supported by Easy Jet, aims to fly an electric 150-seater plane by 2030, to cover relatively short distances such as between Amsterdam and London.
For large airline companies, full electrification, however, is unlikely in the foreseeable future. This is mostly because the energy density of batteries today is significantly too low compared with liquid fuels, especially since weight is a critical parameter in airplane design.36 Nevertheless, Norway aims to fully electrifying all short-haul flights from 2040. Avinor, the Norwegian airport operator, aims to have the first commercial route using electric planes before 2030 (Avinor, 2019).
Energy use and well-to-wheel GHG emissions
Electricity demand and oil displacement
The global EV fleet consumed approximately 58 terawatt-hours (TWh) of electricity in 2018 (Figure 1.6), which is slightly less than total electricity consumed in Switzerland in 2017. Twowheelers continued to account for the largest share (55%) of global electricity demand for EVs in 2018. LDVs are the mode that increased the most in 2018 compared with the previous year and accounted for 24% of power demand for electric vehicles.
Electricity demand for EVs was highest in China in 2018 at 47 TWh, 83% of the total, which is mostly attributable to two-wheelers and public buses. Electricity demand for EVs is over 5 TWh (9% of the total) in Europe and slightly more than 4 TWh in the United States.
Global electricity demand from EVs increased by 17% in 2018, slightly less than in the two previous years. EVs in 2018 continued to account for a small fraction (less than 0.5%) of global total final consumption of electricity.37 Even in China, Norway and the Netherlands, the countries with the highest share of electricity demand from EVs, the percentage is just below 1%.
While consuming 58 TWh of electricity, the global EV stock in 2018 displaced the consumption of approximately 21 million tonnes of oil equivalent (Mtoe) (0.43 million barrels of oil per day) of oil products. The majority of oil displacement is attributable to two-wheelers (60%) followed by LDVs (23%) and buses (18%).
35The first flights were announced for 2022.
36In this regard, electric propulsion in aviation is being actively researched in the case of passenger and cargo drones for short trips and short-distance deliveries in urban areas. Boeing and Airbus have designed demonstration drones. Uber, in association with Boeing, is projecting first commercial flights in 2023. However, there remain technical and regulatory challenges to overcome before passenger drones fly in cities. It is unclear at this point what market segment such services would target and if they can attract and serve a large customer base.
37Note that the actual implication of EV charging on the power grid largely depends on the timing and rapidity of the charging events. This aspect is discussed in Chapter 5, Implications of electric mobility for power systems.
PAGE | 50
IEA. All rights reserved.
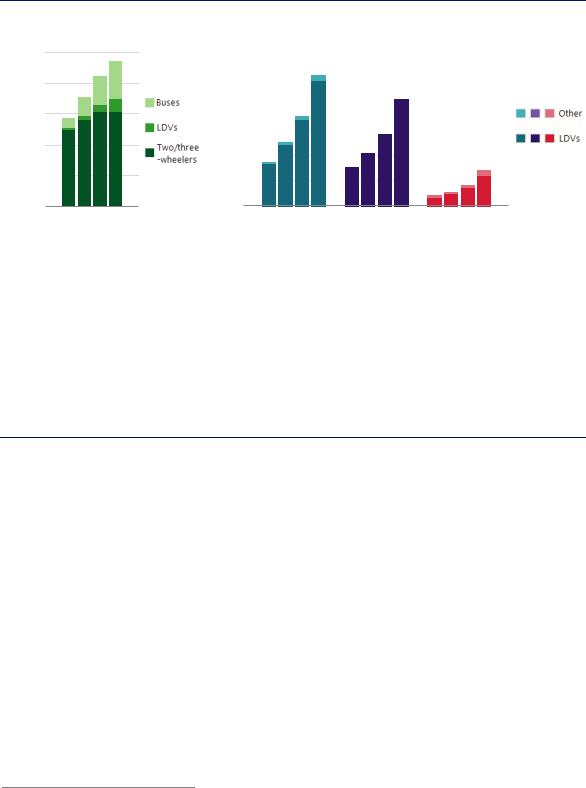
Global EV Outlook 2019 |
1. Status of electric mobility |
Figure 1.6. Electricity demand from EVs by region and type of technology, 2015-18
EV electricity demand (TWh)
50
40
30
20
10
0
China
|
|
|
|
2015 |
2018 |
EV electricity demand (TWh)
6
5
4
3
2
1
0
|
Europe |
|
United States |
Other |
|||||||||
|
|
|
|
|
|
|
|
|
|
|
|
|
|
|
|
|
|
|
|
|
|
|
|
|
|
|
|
|
|
|
|
|
|
|
|
|
|
|
|
|
|
|
|
|
|
|
|
|
|
|
|
|
|
|
|
|
|
|
|
|
|
|
|
|
|
|
|
|
|
|
|
|
|
|
|
|
|
|
|
|
|
|
|
|
|
|
|
|
|
|
|
|
|
|
|
|
|
2015 |
2018 |
2015 |
2018 |
2015 |
2018 |
Notes: Electricity demand by EV mode and by country has been calculated using the following assumptions (where the range indicates the variation across countries). Passenger car: consumption (on road) 0.2-0.24 kWh/km, mileage 9 00020 000 km/year; bus: consumption 1.3-1.8 kWh/km, mileage 25 000-50 000 km/year; two-wheeler: consumption 0.03-0.04 kWh/km, mileage 5 000- 7 300 km/year; truck: consumption 1-1.3 kWh/km, mileage 25 000-70 000 km/year. PHEVs have a share of electric driving of 55% and EV charging losses are 5%.
In this analysis, and only in this analysis, the countries considered are Australia, Austria, Belgium, Brazil, Canada, Chile, China, Denmark, Finland, France, Germany, Iceland, India, Italy, Japan, Korea, Malaysia, Mexico, Netherlands, New Zealand, Norway, Portugal, South Africa, Spain, Sweden, Switzerland, Thailand, United Kingdom and United States. The number of electric vehicles not included in this analysis does not exceed 0.5% of the total stock.
Sources: IEA analysis based on country submissions and the Mobility Model.
China accounts for 83% of the total electricity consumed by the global EV fleet. Two-wheelers are the mode of transport accounting for most of the EV electricity demand.
Well-to-wheel GHG emissions
The 2018 EV stock emitted about 38 million tonnes of carbon-dioxide equivalent (Mt CO2-eq) on a well-to wheel (WTW) perspective. This compares to 78 Mt CO2-eq that an equivalent internal
combustion engine (ICE) fleet would have emitted, leading to net savings from EV deployment of 40 Mt CO2-eq (Figure 1.7).38,39 These savings were achieved through the combination of the
high energy efficiency of the electric powertrain and the carbon intensity of the power grid. The EV fleet in China is the largest contributor to these savings, accounting for about 30 Mt CO2, 77% of the total. This result reflects the large magnitude of the EV stock in China, rather than a significant advantage of EVs over ICE vehicles in terms of WTW CO2 emissions given the high carbon intensity of China’s power generation mix.
38These numbers have been evaluated with a well-to-wheel analysis, which accounts for well-to-tank emissions (upstream emissions due to oil extraction and processing, and to power generation and distribution) and tank-to-wheel emissions (tailpipe emissions). An analysis of life-cycle GHG emissions that takes into account the emissions due to the electric car manufacturing process is in Chapter 4.
39Emissions from the EV fleet are calculated by multiplying the electricity consumption by the average CO2 intensity of electricity generation in each country. The avoided emissions are determined as the CO2 that would have been emitted if the EV fleet was powered by ICE vehicles with a fuel economy representative of the country average.
PAGE | 51
IEA. All rights reserved.