
Учебное пособие 2163
.pdf
Russian Journal of Building Construction and Architecture
be considered good values for use for architectural purposes. Anyway, the reason for choosing a slightly higher ratio of cement in mix designs is balancing of the compressive strength. According to the results of bending, the lowest bending strength is associated with the specimen containing only the glass aggregate. Although the flexural strengths of the M5 and M6 specimens with good aesthetic appearance are somewhat lower than those of the control specimen, they are generally good values (6.73 and 6.58, respectively). In the impact test, the lowest value (31.81) is related with M6, i.e. the specimen containing both glass aggregate and the maximum amount of glass powder. However, the ultimate energy value of M5, which is similar to this specimen, is very close to the result for the other specimens.
In the heat test examination, the minimum temperature increase of 18.2 was observed in M5. The heat transfer ability of all the specimens containing glass aggregate and glass powder were reduced compared to that of the control specimen. Among them, M5, which contains glass aggregate and glass powder together, exhibited a lower heat conduction behavior than the others. As a result, for this study the ratio of the glass powder to the glass aggregate of 1/3 as selected in M5 seems appropriate. It is also suggested that the self-assembly tools employed to study impact and heat permeability be used in the examination of construction building materials, because they have been found to give meaningful insights into these matters.
References
1.Kılıçoğlu C., and S. Çoruh. Atık Cam ve Yüksek Fırın Cürufu Kullanılarak Hazırlanan Betonlarda AlkaliSilika Reaksiyonu in Ulusal Mühendislik Jeolojisi Sempozyumu, KTÜ, Trabzon, 2015.
2.Anon VIII. Beş Yıllık Kalkınma Planı İçme Suyu, Kanalizasyon, Arıtma Sitemler ve Katı Atık Denetimi İhtisas Komisyonu, Katı Atık Denetimi Alt Komisyonu Raporu in D. P. T.Yayınlan, Ankara, 2001.
3.Kavas T., Çelik M., and Evcin A., Cam atıklarının çimento üretiminde katkı maddesi olarak kullanılabilirliğinin araştırılması, 5. Endüstriyel Hammaddeler Sempozyumu, İzmir, 2004, pp. 114––119.
4.Kara P. Recycling of Glass Wastes in Latvia–Its Application as Cement Substitute in Self-Compacting Concrete. Journal of Sustainable Architecture and Civil Engineering, 2014, no. 6 (1), pp. 42––49.
5.Rakshvir M., and Barai S. V. Studies on recycled aggregates-based concrete. Waste Management & Research, 2006, no. 24 (3), pp. 225––233.
6.Siddique R. Waste materials and by-products in concrete. 2007: Springer Science & Business Media.
7.Corinaldesi V., e. a. Reuse of ground waste glass as aggregate for mortars. Waste Management, 2005, no. 25(2), pp. 197––201.
8.Ismail Z. Z., and Al-Hashmi E. A. Recycling of waste glass as a partial replacement for fine aggregate in concrete. Waste management, 2009, no. 29 (2), pp. 655––659.
9.Topcu I. B., and Canbaz M. Properties of concrete containing waste glass. Cement and concrete research, 2004, no. 34 (2), pp. 267––274.
40
Issue № 4 (44), 2019 |
ISSN 2542-0526 |
10.Shao Y., e. a. Studies on concrete containing ground waste glass. Cement and Concrete Research, 2000, no. 30 (1), pp. 91––100.
11.Shi C., e. a. Characteristics and pozzolanic reactivity of glass powders. Cement and Concrete Research, 2005, no. 35 (5), pp. 987––993.
12.Schwarz N., Cam H., and Neithalath N. Influence of a fine glass powder on the durability characteristics of concrete and its comparison to fly ash. Cement and Concrete Composites, 2008, no. 30 (6), pp. 486––496.
13.Tung-Chai L., Chi-Sun P., and Hau-Wing W. Management and recycling of waste glass in concrete products: Current situations in Hong Kong Resources. Conservation and Recycling, 2013, no. 70 (1), pp. 25––31.
14.Ling T.-C., and Poon C.-S. Properties of architectural mortar prepared with recycled glass with different particle sizes. Materials & Design, 2011, no. 32 (5), pp. 2675––2684.
15.Yildirim K., and Sümer M. Effects of sodium chloride and magnesium sulfate concentration on the durability of cement mortar with and without fly ash. Composites Part B: Engineering, 2013, no. 52, pp. 56––61.
16.Zhang W., Zakaria M., and Hama Y. Influence of aggregate materials characteristics on the drying shrinkage properties of mortar and concrete. Construction and building materials, 2013, no. 49, pp. 500––510.
17.Khattab M. M. Effect of gamma irradiation on polymer modified white sand cement mortar composites. Journal of Industrial and Engineering Chemistry, 2014, no. 20 (1), pp. 1––8.
18.Ambily P., e. a. Studies on ultra high performance concrete incorporating copper slag as fine aggregate. Construction and Building Materials, 2015, no. 77, pp. 233––240.
19.EN, T., 196-1. Methods of testing cement–Part 1: Determination of strength. European Committee for standardization, 2005. 26.
20.Idir R., Cyr M., and Tagnit-Hamou A. Pozzolanic properties of fine and coarse color-mixed glass cullet. Cement and Concrete Composites, 2011, no. 33 (1), pp. 19––29.
21.Bilim C., and Atiş C. D. Alkali activation of mortars containing different replacement levels of ground granulated blast furnace slag. Construction and Building Materials, 2012, no. 28 (1), pp. 708––712.
22.Pereira-de-Oliveira L. A., Castro-Gomes J. P., and Santos P. M. The potential pozzolanic activity of glass and red-clay ceramic waste as cement mortars components. Construction and Building Materials, 2012, no. 31, pp. 197––203.
23.Xu S., Gao L., and Jin W. Production and mechanical properties of aligned multi-walled carbon nanotubesM140 composites. Science in China Series E: Technological Sciences, 2009, no. 52 (7), pp. 2119––2127.
24.Sun W., e. a. Damage and damage resistance of high strength concrete under the action of load and freezethaw cycles. Cement and Concrete Research, 1999, no. 29 (9), pp. 1519––1523.
25.Yahaghi J., Muda Z. C., and Beddu S. B. Impact resistance of oil palm shells concrete reinforced with polypropylene fibre. Construction and Building Materials, 2016, 123, pp. 394––403.
26.Dehghanpour H., and Yilmaz K. Mechanical and Impact Behavior on Recycled Steel Fiber Reinforced Cementitious Mortars. Russian Journal of Building Construction and Architecture, 2018, no. 3 (39), pp. 67––84.
27.Nili M., and Afroughsabet V. The effects of silica fume and polypropylene fibers on the impact resistance and mechanical properties of concrete. Construction and Building Materials, 2010, no. 24 (6), pp. 927––933.
28.Dehghanpour H., and Yılmaz K. ABAQUS Modeling and Investigation of Thermal Conductivity of NanoPorous Alumina Coating, in 1st International Symposium on Light Alloys and Composite Materials (ISLAC’18). 2018, ISLAC'18, pp. 22––23.
41

Russian Journal of Building Construction and Architecture
29.Bomeni I., e. a. Ceramic with potential application of ngwenfon alluvial clays (noun, west cameroon) in building construction: Mineralogy, physicochemical composition and thermal behaviour. Construction and Building Materials, 2018, 182, pp. 493––503.
30.Pavelek M., Prajer M., and Trgala K. Static and dynamic thermal characterization of timber frame/wheat (Triticum Aestivum) chaff thermal insulation panel for sustainable building construction. Sustainability, 2018, no. 10 (7), pp. 2363.
31.Dehghanpour H., and Yılmaz K. Simulation and Characterization of Thermal Conductivity of Nano Silica Based Aerogel, in 1st International Symposium on Light Alloys and Composite Materials (ISLAC’18), 2018, ISLAC'18, pp. 515––516.
32.Sharifi Y., Afshoon I., and Firoozjaie Z. Fresh properties of self-compacting concrete containing ground waste glass microparticles as cementing material. Journal of Advanced Concrete Technology, 2015, no. 13(2), pp. 50––66.
33.Ali E. E., and Al-Tersawy S. H. Recycled glass as a partial replacement for fine aggregate in self compacting concrete. Construction and Building Materials, 2012, no. 35, pp. 785––791.
34.Tuaum A., Shitote S., and Oyawa W. Experimental Study of Self-Compacting Mortar Incorporating Recycled Glass Aggregate. Buildings, 2018, no. 8 (2), pp. 15.
35.Nunes S., e. a. Mixture design of self-compacting glass mortar. Cement and Concrete Composites, 2013, no. 43, pp. 1––11.
36.Properties A. S. D. o. M. Standard test methods for flexural properties of unreinforced and reinforced plastics and electrical insulating materials. 1997. American Society for Testing Materials.
42
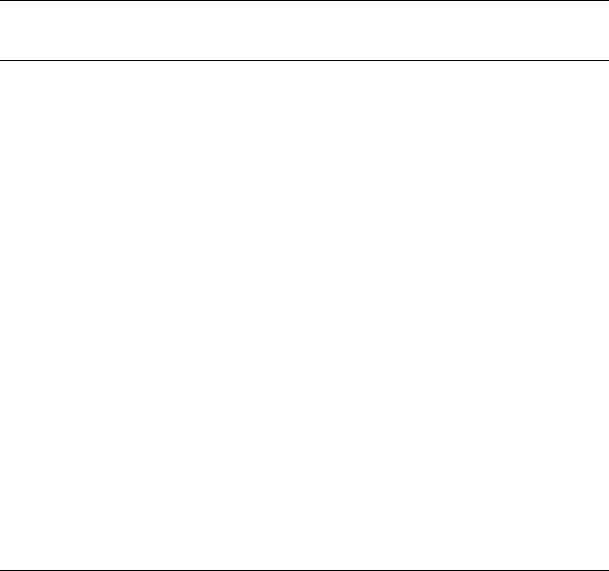
Issue № 4 (44), 2019 |
ISSN 2542-0526 |
DESIGNING AND CONSTRUCTION OF ROADS,SUBWAYS,
AIRFIELDS,BRIDGES AND TRANSPORT TUNNELS
DOI 10.25987/VSTU.2019.44.4.003
UDC625.855.3
Yu. G. Borisenko 1, S. O. Kazaryan 2
FEATURES OF THE STRESS STRAIN OF CERAMSITE POWDER MODIFIED
SMA PAVEMENTS
North-Caucasus Federal University 1, 2
Russia, Stavropol
1 PhD in Engineering, Assoc. Prof. of the Dept. of Construction
2 Senior Lecturer of the Dept. of Construction, e-mail: sam23otr@mail.ru
Statement of the problem. The task of improving the performance characteristics of crushed stone-mastic asphalt concrete by modifying them with porous powder materials, using the example of expanded clay powder, is considered.
Results. As a result of experimental studies features of the deformative behavior of the proposed compositions were identified at different operating temperatures. The graphs of dependences of the impact of expanded clay powder on the viscosity and relaxation time of the stresses of crushed stone-mastic asphalt concrete were obtained.
Conclusions. It was established experimentally that crushed stone-mastic asphalt concrete modified with expanded clay powder has a higher heat resistance, shear resistance and crack resistance than traditional compositions with stabilizing additives. Increasing these properties leads to an increase in the service life of road surfaces.
Keywords: stone mastic asphalt concrete, road pavement, expanded clay powder, rheology, viscosity, stress relaxation, Viatop-66.
Introduction. Asphalt concrete is an elastic viscous plastic body which is subject to a whole host of factors, e. g., transport loads, heat, cooling, humidity, etc. Calculations for this type of structures commonly rely on the strength of asphalt concrete and its transport load resistance. Possible shear deformations in hot season or temperature-induced cracks in winter season are not necessarily given any consideration. The reason why these should be is that along with traffic load, these temperature deformations are one of the major factors causing pavement to
© Borisenko Yu. G., Kazaryan S. O., 2019
43

Russian Journal of Building Construction and Architecture
fail prematurely. In order for temperature effects to be considered in pavement calculations, there has to be a clear understanding of how asphalt concrete functions under transport load both at positive temperatures and under cooling ultimately leading to tensile strains and deformations [9]. These properties of asphalt concrete largely depend on the behavior of the binder under varying conditions. As the temperature of bitumen goes up, shear resistance and strength of asphalt concrete drop due to a reduction of physical and chemical links between mineral particles and a bitumen binder [3, 12].
1. Viscosity and strain relaxation in asphalt concrete. In order for asphalt concrete to function properly under varying operational conditions, it is essential that the following conditions are met: sufficient deformation resistance at high summer temperatures (i. e., thermal resistance) and sufficient deformation capacity at low temperatures (i. e., a high crack resistance). Unless these are met, road pavement failures are rather common to occur. Most asphalt concrete pavements in this country these days do not meet the above requirements. The problem can be dealt with by increasing crack resistance of pavements due to a larger internal friction coefficient tgφ and cohesion of mineral grains and that generated by bitumen links. An increase in crack resistance of pavements can be due to use of fillers with a developed rough surface to increase internal friction and cohesion between stone material particles [1––2, 4––8].
Cohesion generated by bitumen links has a direct effect on the likelihood of plastic deformations at high operational temperatures. Larger bitumen activity and viscosity can be achieved by introducing various modifying additives [16––20]. However, if bitumen should be maximum viscous at high temperatures, it should be minimum at negative temperatures as it is under these particular conditions that significant stretching strains occur in asphalt concrete pavements causing cracks, a lower freeze resistance and, therefore, failure of a material. It is necessary that bitumen was maximum viscous at high temperatures and also had a high plasticity at low temperatures. In practice, this seems rather challenging. Therefore in order for these conditions to be met and compositions with most optimal properties to be obtained, the appropriate materials are to be employed. One of them are porous mineral fillers modifying and structuring bitumen in the process of their interaction [10––11, 13––15].
For high-quality evaluation of operating properties and durability of materials, the stressstrain behavior of asphalt concrete should be considered at varying operational temperatures, which is best described in rheology, i. e. the flow behavior study.
One of the most important properties of asphalt concrete as an elastic-viscous-plastic material is strain relaxation. Strain relaxation is uncontrolled decrease (dispersion) of strains in time which
44
Issue № 4 (44), 2019 |
ISSN 2542-0526 |
is caused by an internal flow of a deformed body (under stable deformation). As the temperature rises, viscosity drops accompanied by rising strain relaxation time for asphalt concrete. Thus relaxation time for asphalt concrete largely depends on the viscosity and temperature.
Viscous or elastic properties in asphalt concrete depend on the load impact and strain relaxation time ratio. If load impact time is very short compared relaxation time, a material functions as an ideally plastic one. If it is long compared to relaxation time, a material displays the properties of a viscous liquid [3, 9, 12].
At negative temperatures asphalt concrete becomes maximum viscous. Under these conditions relaxation time is more than common load impact times. In this case asphalt concrete acts as an elastic material, which results in an increase in its fragility and tensile strength limit Rp , which makes crack more likely to occur. If emerging strains are completely or significantly relaxed by the end of a period of a sharp temperature drop, cracks are least likely to appear. Therefore in the winter season it is recommended that relaxation time and viscosity of asphalt concrete is reduced.
At high temperatures viscosity of asphalt concrete is at its lowest, which effectively causes a reduction in strain relaxation times which is comparable or significantly smaller than load impact times, which leads to irreversible plastic deformations. Thus it is necessary that relaxation time and viscosity of asphalt concrete is at its highest.
In this study the effect of a high-dispersion ceramsite powder on such rheological parameters of SMA asphalt concrete mixes as viscosity and strain relaxation times. The compositions of SMA asphalt concrete were modified with the ceramsite powder that was used instead of a stabilizing additive. In order to identify the efficiency of the suggested materials, the standard SMA asphalt concrete was compared with Viatop stabilizing additives.
2. Study of rheological properties of SMA. Rheological properties of the investigated SMA were researched according to the method in [9]. Rheological parameters were determined for SMA-10 and SMA-15 by testing the sample cylinders with the diameter and height (71.5±1.5) mm at different temperatures. In order to calculate rheological characteristics, geometric parameters (diameter and height) were measured before and after testing and tensile strength limits were also fixed. The rate of the press plate motion during the test was assumed to be = 0.005 cm/sec. Dependencies of the rheological characteristics were investigated at operating temperatures ranging from –10 to +60 оС. The temperature of +60 оС was chosen as, according to natural observations, in the southern parts of the country the pavement tem-
45

Russian Journal of Building Construction and Architecture
perature could reach this value and during the hot season it can hold for a few hours a day and –10 оС is the temperature at which asphalt concrete functions almost as an elastic body. All of the applied temperatures modeled the operation of asphalt concrete for different states of a material: elastic, elastic-viscous, viscous-plastic and plastic stages.
Rheological parameters were calculated based on the following formulas: –– viscosity coefficient:
м 400R h12d 2h ,
where R is the tensile strength limit, МPа; h1 is the height of the sample before the test, сm; h is a difference between the heights of the sample before and after the test (Δh = h1 – h2), сm; h is a difference between the diameters before and after the test (Δd = d1 – d2), cm;
–– relaxation time:
400h1dh22 .
Rheological parameters were identified for SMA-10 and SMA-15 modified with ceramsite powder. For comparison standard SMA with a Viatop-66 stabilizing additive were tested. The dependencies of changes in the viscosity coefficient м on the temperature Т are presented in Fig. 1 and 2 for SMA-10 and SMA-15 respectively.
Viscosity coefficient ηм,∙10-6
350
300
250
200
150
100
50
0
-10 |
0 |
10 |
20 |
30 |
40 |
50 |
60 |
|
|
|
|
|
Temperature, T, °С |
|
|
|
CompositionСостав № 1 1 |
SMA-10 with a Viatop-66 additive |
|
||||
|
|
|
|
||||
|
CompositionСостав № 2 2 |
SМА-10 modified with the ceramsite powder |
|
|
Fig. 1. Dependencies of changes in the viscosity coefficient м on the temperature Т for SMA-10
The dependencies of changes in the relaxation time on the temperature Т are presented in Fig. 3 and 4 for SМА-10 and SМА-15 respectively.
46
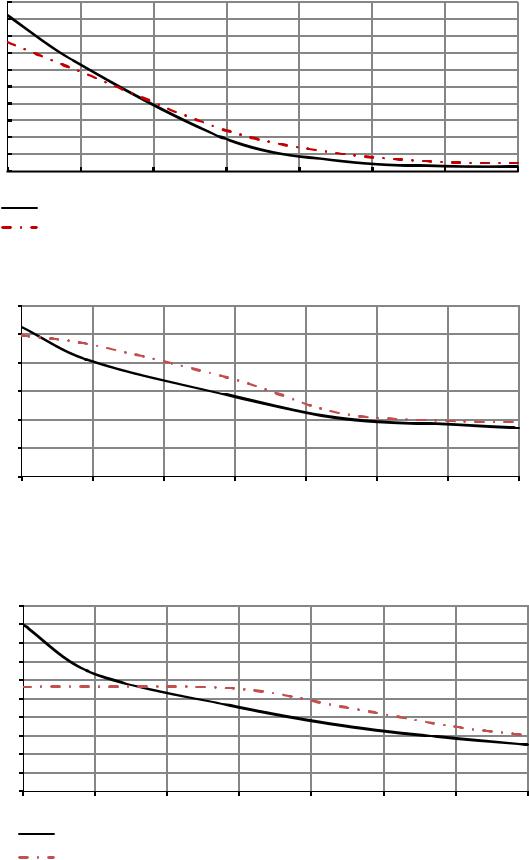
Issue № 4 (44), 2019 |
ISSN 2542-0526 |
Viscosity coefficient ηм,∙10-6
200
180
160
140
120
100
80
60
40
20
0
-10 |
0 |
|
10 |
20 |
30 |
40 |
50 |
60 |
|
|
|
|
|
|
|
Теmperature, T, °С |
|
|
CompositionСостав № 1 |
1 |
|
SMA-15 with a Viatop-66 additive |
|
|
||
|
|
|
|
|
||||
|
CompositionСостав № 2 |
2 |
|
SMA-15 modified with the ceramsite powder |
|
|||
|
|
|
|
|
|
|
|
|
Fig. 2. Dependencies of changes in the viscosity coefficient м on the temperature Т for SMA-15
Relaxation time, θ, с
12000
10000
8000
6000
4000
2000
0
-10 0
CompositionСостав № 1 1
CompositionСостав № 2 2
10 |
20 |
30 |
40 |
50 |
60 |
SМА-10 with a Viatop-66 additive
SМА-10 modified with the ceramsite powder
Relaxation time, θ, с
Fig. 3. Dependencies of changes in the relaxation time on the temperature Т for SМА-10
10000
9000
8000
7000
6000
5000
4000
3000
2000
1000
0
-10 |
0 |
|
10 |
20 |
30 |
40 |
50 |
60 |
|
|
|
|
|
|
|
Теmperature, T, °С |
|
|
CompositionСостав № 1 |
1 |
SМА-15with a Viatop-66 additive |
|
|
|||
|
|
|
|
|||||
|
Composition 2 |
SМА-15 modified with the ceramsite powder |
|
|||||
|
Состав № 2 |
|
|
|
|
|
|
|
Fig. 4. Dependencies of changes in the relaxation time on the temperature Т for SМА-15
47

Russian Journal of Building Construction and Architecture
According to the resulting dependencies (Fig. 1––4), SМА-10 and SМА-15 modified with the ceramsite powder have a lower viscosity and a shorter strain relaxation time at low temperatures than the compositions with a Viatop-66 stabilizing additive. Thus the modified compositions have a larger deformability and are less fragile and thus more crack-resistant.
It was found that at high operating temperatures the viscosity of SМА-10 and SМА-15 modified with the ceramsite powder is higher than that of the standard SМА-10 and SМА-15 compositions (Fig.1,2). Asthe viscosity ofasphaltconcreteincreases,sodoesitsheatandshearresistance.
According to the dependencies (Fig. 3, 4), strain relaxation time of modified SMA is longer than for the standard compositions making plastic deformations less likely at high operating temperatures.
Conclusions. Through the course of the studies of rheological characteristics of SMA asphalt concrete the following conclusions were made:
––at negative operating temperatures SMA modified with ceramsite powder are highly crackresistant and less fragile than standard SMA with a Viatop-66 stabilizing additive;
––at high operating temperatures they have a high shear and heat resistance as well as plastic deformation resistance.
We argue that an increase in the rheological and operational properties of modified SMA is due to SMA asphalt concrete ceramsite powder mixes.
Besides, porous powder materials (e. g., high-dispersion sieves of ceramsite crushing) included into asphalt concrete and bitumen and mineral compositions allow the heat conductivity of materials and thus temperature strains in pavements to be reduced, which causes a significant freeze and crack resistance of materials.
According to the study, due to the topography and microstructure of a surface as well as interaction with a bitumen filler, use of porous powder materials contributes to improving operational and rheological characteristics of SMA and thus longer life cycles of pavements.
References
1.Ageikin V. N., Svintitskikh L. E., Shabanova T. N., Klyusov A. A. Issledovanie vliyaniya vspuchennogo vermikulitovogo peska na svoistva bitumnykh kompozitsii i asfal'tobetonov [Investigation of the influence of expanded vermiculite sand on the properties of bitumen compositions and asphalt concretes]. Stroitel'nye materialy, 2003, no. 7, pp. 40––42.
2.Aminov Sh. Kh., Strugovets I. B., Khannanova G. G., Nedoseko I. V., Babkov V. V. Ispol'zovanie piritnogo ogarka v kachestve mineral'nogo napolnitelya v asfal'tobetonakh [The use of pyrite stub as a mineral filler in asphalt concrete]. Stroitel'nye materialy, 2007, no. 9, pp. 42––43.
48
Issue № 4 (44), 2019 |
ISSN 2542-0526 |
3.Boguslavskii A. M., Boguslavskii A. A. Osnovy reologii asfal'tobetona [Fundamentals of asphalt concrete rheology]. Moscow, Vysshaya shkola Publ., 1972. 200 p.
4.Borisenko Yu. G., Soldatov A. A., Yashin S. O. Bitumno-mineral'nye kompozitsii, modifitsirovannye vysokodispersnymi otsevami drobleniya keramzita [Bituminous-mineral compositions modified by highly dispersed screenings of expanded clay crushing]. Stroitel'nye materialy, 2009, no. 1, pp. 62––63.
5.Budyanskaya L. A., Makhrov E. Yu., Efa A. K. [Macadamized asphalt concrete in Western Siberia: experience of application]. Trudy 4 Mezhdunarodnoi nauchno-tekhnicheskoi konferentsii «Sovremennye tekhnologii ustroistva i soderzhaniya dorozhnogo pokrytiya» [Proc. 4th International scientific and technical conference "Modern technologies of construction and maintenance of road surface"]. Zvenigorod, 2004. 324 p.
6.Kiryukhin G. N., Smirnov E. A. Pokrytiya iz shchebenochno-mastichnogo asfal'tobetona [Coatings of crushed stone-mastic asphalt concrete]. Moscow, Elit Publ., 2009. 176 p.
7.Vysotskaya M. A., Kuznetsov D. K., Fedorov M. Yu. Otsenka kachestva bitumomineral'nykh kompozitov s primeneniem poristykh napolnitelei [Assessment of the quality of bitumen-mineral composites using porous fillers]. Dorogi i mosty, 2012, no. 27, pp. 241––250.
8.Vysotskaya M. A., Kuznetsov D. K., Barabash D. E. Osobennosti strukturoobrazovaniya bitumomineral'nykh kompozitsii s primeneniem poristogo syr'ya [Features of structure formation of bitumen-mineral compositions using porous raw materials]. Stroitel'nye materialy, 2014, no. 1––2, pp. 68––71.
9.Gezentsvei L. B., ed. Dorozhnyi asfal'tobeton [Road asphalt concrete]. Moscow, Transport Publ., 1985. 350 p.
10.Grusho-Novitskaya A. O., Yartsev V. P. Vliyanie dispersnosti i kolichestva otkhodov keramzita na ekspluatatsionnye kharakteristiki bituma BN 90/10 [Effect of dispersion and amount of expanded clay waste on performance characteristics of bitumen bn 90/10]. Vestnik BGTU im. V. G. Shukhova, 2003, no. 5, pp. 28––31.
11.Gridchin A. M., Korotaev A. P., Yadykina V. V., Kuznetsov D. A., Vysotskaya M. A. Dorozhnye kompozity na osnove dispersnogo vspuchennogo perlita [Road composites based on dispersed expanded perlite].
Stroitel'nye materialy, 2009, no. 5, pp. 42––44.
12.Mikhailo A. A., Kalgin Yu. I. [Rheological characteristics of cold asphalt concrete based on modified liquid bitumen]. Trudy nauchnoi konferentsii «33 Ogarevskie chteniya». Ch. 2. Estestvennye i tekhnicheskie nauki
[Proc. of the scientific conference «33 Ogarev readings». Part 2. Natural and technical Sciences]. Saransk, Izd-vo Mordovskogo universiteta, 2005, pp. 274––280.
13.Korotaev A. P. Povyshenie kachestva asfal'tobetona za schet ispol'zovaniya poristogo mineral'nogo poroshka. Diss. kand. tekhn. nauk [Improving the quality of asphalt concrete through the use of porous mineral powder. Cand. eng. sci. diss.]. Belgorod, 2009. 169 p.
14.Svintitskikh L. E., Shabanova T. N., Klyusov A. A., Ageikin V. N. Vliyanie dispersnosti vspuchennogo vermikulita na svoistva bitumnogo vyazhushchego i asfal'tobetona [Effect of dispersion of expanded vermiculite on properties of bitumen binder and asphalt concrete]. Stroitel'nye materialy, 2004, no. 9, pp. 32––33.
15.Soldatov A. A., Borisenko Yu. G. Struktura poverkhnosti poristykh poroshkov na osnove otsevov drobleniya keramzita i ikh adsorbtsionnaya aktivnost' [Surface structure of porous powders based on expanded clay crushing screenings and their adsorption activity]. Stroitel'nye materialy, 2011, no. 6, pp. 36––38.
16.Ameri M., Mohammadi R., Vamegh M., Molayem M. Evaluation the effects of nanoclay on permanent deformation behavior of stone mastic asphalt mixtures. Construction and Building Materials, 2017, no. 156, pp. 107––113.
49