
Учебное пособие 2110
.pdf
Russian Journal of Building Construction and Architecture
5.Buzinov S. N., Mikhaylovskii A. A., Solov'ev A. N., Parfenov V. I. Shchelkovskoe podzemnoe khranilishche gaza: problemy, resheniya i perspektivy [Shchelkovo underground gas storage: problems, solutions and prospects]. Transport i khranenie gaza, 2003. 59 p.
6.Vlasov S. V., Ostrovskii V. R., Tutnov I. A., Shpara I. A. Razvitie metodologii veroyatnostnogo analiza bezopasnosti dlya preduprezhdeniya yavlenii razrushitel'nogo i pozharoopasnogo kharaktera na energeticheskikh kompleksakh podzemnogo khraneniya gaza [Development of the methodology of probabilistic safety analysis to prevent the phenomena of destructive and fire-dangerous nature at the energy complexes of underground gas storage]. Neft', gaz i biznes, 2012, no. 12, pp. 44—53.
7.Gershanovich G. G., Kalmykov E. F. Metodicheskie ukazaniya po optimizatsii tekhniko-ekonomicheskikh pokazatelei podzemnykh khranilishch gaza [Methodical instructions on optimization of technical and economic indicators of underground gas storage facilities]. Moscow, VNIIEgazprom, 1983. 45 p.
8.Gurbanov A. N. Povyshenie effektivnosti tekhnologii podgotovki gaza k transportu na podzemnykh gazokhranilishchakh Azerbaidzhana [Improving the efficiency of gas treatment technology for transport at underground gas storage facilities in Azerbaijan]. Naftogazova energetika, 2014, no. 2 (22), pp. 57––62.
9.Dudin S. M., Nekrasov V. O., Zemenkov Yu. D. [Physical and mathematical modeling of technological modes of transportation and storage of hydrocarbon media in pipeline systems]. Neft' i gaz: otdel'nyi vypusk Gornogo informatsionno-analiticheskogo byulletenya (nauchno-tekhnicheskogo zhurnala) [Oil and gas: separate issue of Mining information and analytical Bulletin (scientific and technical journal)]. Moscow, Gornaya kniga Publ., 2013, pp. 53––62.
10.Ermilov O. M., Remizov V. V., Shirkovskii A. I., Chugunov L. S. Fizika plasta, dobycha i podzemnoe khranenie gaza [Reservoir physics, production and underground gas storage]. Moscow, Nauka Publ., 1996, 541 p.
11.Buzinov S. N., Voronov S. A., Dudnikova Yu. K. e. a. [Intellectualization of underground gas storage processes]. Tezisy dokladov 3-i mezhdunarodnoi nauchno-tekhnicheskoi konferentsii “PKhG: nadezhnost' i effektivnost'” [Abstracts of the 3rd international scientific and technical conference “UGS: reliability and efficiency”]. Moscow, Gazprom VNIIGAZ Publ., 2011. P. 27.
12.Istoriya nachinalas' tak [The story began]. Vestnik GAZPROM PKhG, 2015, no. 1 (65), pp. 1––3.
13.Kazaryan V. A. Podzemnoe khranenie gazov i zhidkostei [Underground storage of gases and liquids]. Izhevsk, NITs “Regulyarnaya i khaotichnaya dinamika”, 2006. 432 p.
14.Kiselev A. I., Soldatkin G. I. Problemy optimizatsii razvitiya i ekspluatatsii PKhG [Problems of optimization of UGS development and operation]. Transport i khranenie gaza, 1979, no. 1, pp. 6––10.
15.Lur'e M. V., Didkovskaya A. S., Varchev D. V., Yakovleva N. V. Podzemnoe khranenie gaza [Underground gas storage]. Moscow, Neft' i gaz Publ., 2004. 172 p.
16.Medvedeva O. N., Ivanov A. I. Osnovy szhiganiya gazovogo topliva [Basics of gas fuel combustion]. Saratov, ID “Rait-Ekspo”, 2016. 130 p.
17.Podzemnoe khranenie gaza. Problemy i perspektivy: sbornik nauchnykh trudov [Underground gas storage. Problems and prospects: collection of scientific works]. Moscow, VNIIGAZ Publ., 2008. 477 p.
18.Shipovalov A. N., Zemenkova M. Yu., Shpilevoi V. A. e. a. Razrabotka energoresursosberegayushchikh tekhnologii pri ekspluatatsii PKhG gazotransportnoi sis-temy [Development of energy-saving technologies in the operation of UGS gas transportation system]. Sovremennye problemy nauki i obrazovaniya, 2015, no. 2. Available at: http://www.science-education.ru/ru/article/view?id=21717
30
Issue № 1 (41), 2019 |
ISSN 2542-0526 |
19.Vlasov S. V., Eshchenko I. V., Silant'ev L. G. e. a. Sovershenstvovanie informatsionnykh i tekhnicheskikh sredstva diagnostiki i monitoringa promyshlennoi bezopasnosti PKhG [Improvement of information and technical means of diagnostics and monitoring of industrial safety of UGS]. Gazovaya promyshlennost', 2013, no. 6, pp. 78––82.
20.Sukharev M. G., Starovskii V. R. Rezervirovanie sistem magistral'nykh truboprovodov [Redundancy of trunk pipeline systems]. Moscow, Nedra Publ., 1987. 168 p.
21.Energeticheskaya strategiya Rossii do 2035 goda [Russia's energy strategy until 2035]. Moscow, Ministerstvo Energetiki RF Publ., 2015. Available at: http://www.energystrategy.ru/ab_ins/source/ES2035_09_2015.pdf
22.Çetin Demirel Nihan, Demirel Tufan, Deveci Muhammet, Vardar Gökhan. Location Selection for Underground Natural Gas Storage Using Choquet Integralю Journal of Natural Gas Science and Engineering, 2017, vol. 45, pp. 368—379.
23.Zhu Quezhi, Duan Peixia, Wang Hongju e. a. Current Situations and Future Development of Oil and Gas Pipelines in the World. Oil Gas Storage Transp, 2015, Vol. 34, no. 12, pp. 1262—1266.
24.Ding Guosheng. Demand and Challenges for Underground Gas Storages in China. Nat Gas Ind, 2011, vol. 31, no. 12, pp. 90 — 93.
25.Dreyer W. Underground Storage of Oil and Gas in Salt Deposits and Other Non-Hard Rocks. New York, Hoisted Press, 1982. 207 p.
26. Zheng Dewen, Xu Hongcheng, Wang Jieming e. a. Key Evaluation Techniques in the Process of Gas Reservoir Being Converted into Underground Gas Storage. Petroleum Exploration and Development, 2017, vol. 44, no. 5, pp. 840 — 849.
27.Lu J., Suping Z. China’S Natural Gas Exploration and Development Strategies Under the New Normal. Natural Gas Industry B., 2015, vol. 2, no. 6, pp. 473—480.
28.Remizov V. V., Rezunenko V. I., Kazaryan V. A. Prospects of Underground Gas Storage Construction in Rock Salt Deposits of Russia. Meeting of SMRI. Cracow, 1997, pp. 475—480.
29.Ding Guosheng, Li Chun, Wang Jieming. e. a. The Status Quo and Technical Development Direction of Underground Gas Storage in China. Nat Gas Ind, 2015, vol. 35, no. 11, pp. 107—112.
30. Lawal K. A., Ovuru M. I., Eyitayo S. I. e. a. Underground Storage As a Solution for Stranded Associated Gas in Oil Fields. Journal of Petroleum Science and Engineering, 2017, vol. 150, pp. 366—375.
31. Žlender, B., S. Kravanja Cost Optimization of the Underground Gas Storage. Engineering Structures, 2011, vol. 33, no. 9, pp. 2554—2562.
31

Russian Journal of Building Construction and Architecture
DOI 10.25987/VSTU.2018.41.1.003
UDC 681.121.8 : 553.981
O. A. Gnezdilova1
AUTOMATED REGISTRATION OF SMALL-SCALE NATURAL GAS CONSUMPTION USING A NEW SOFTWARE PRODUCT
Moscow State Architectural University
Russia, Moscow, e-mail: gnezdilovakgtu@mail.ru, tel.: +7-903-873-89-16 1PhD in Engineering, Assoc. Prof. of the Dept. of Heat and Gas Supply and Ventilation
Statement of the problem. A new developed software product for measuring small-scale consumption of natural gas is described.
Results and conclusions. The major parameters of the developed software for measuring small-scale consumption of natural gas are considered. The software would allow the processing and visualization of incoming data in order to determine the linear velocity of gas particles in the swirl nuclear as well as to evaluate the effect of the weighed phase on changes in the static and dynamic pressure.
The obtained results of the research and software can be applied in energy supply and energysaving systems in gas industry and communal household services. Unlike similar mainstream devices, visualization of changes in the gas pressure in the device would allow one to get extra information on small-scale consumption of the measured gas environment and an increase in the sensitivity of the device.
Keywords: natural gas consumption, automated registration systems, registration software, energy consumption, energy saving, communal household services.
Introduction. This article looks at a new automated system of small-scale gas consumption. An application range of automated systems of commercial gas consumption is for all natural gas users. Automated systems have a centralized structure including a lower level, i.e. controlling stations, and an upper level, i.e. a central dispatcher service with a network of automatic switchboards [10].
Automated management systems encompass gas-distribution stations, gas-controlling centres in highand medium-pressure networks, gas-regulating stations supplying circular and lateral pipelines (with the consumption of over 1000 m3/h) and gas-regulating stations of residential areas. Automatic management systems include information functional subsystems for highspeed control of technological gas distribution.
© Gnezdilova O. A., 2019
32
Issue № 1 (41), 2019 |
ISSN 2542-0526 |
Generally, a gas flowing in pipelines is non-stationary, which is largely due to non-stationary gas consumption.
Hydraulic processes in low-pressure pipelines should be used to describe flow distribution in an approximated quasi-stationary statement of a task. This is due to disappearing small time derivatives of a volumetric consumption [7]. Moreover, a gas distribution mode, as it is quasistationary, depends on the time of a system functioning based on hours of the day, week, month, etc. ordering the inclusion of various loads. Time is a parameter in this case.
1. Description of the software for small-scale natural gas consumption. For high-speed control, analysis of technological measurement of gas consumption, formation and transmission of a signal, there should be periodic measurements and control of the functioning parameters of no more than 5 sec [10].
The function of the software is to develop processing and visualization of incoming data to identify the linear speed of gas particles in a swirl nuclear as well as to evaluate the influence of the weighed phase on changes in the static and dynamic pressure.
Mathematical description of an aerodynamic swirl flow and comparison of the fields of axial and rotational velocities prove that kinematic similarity of the internal swirl flows can be determined with two major dimensionless parameters, i.e. the integral Ф* and local tg . It is known that these parameters are homogeneously connected. This allows this connection to be used to summarize the experimental data. The parameter Ф* is most commonly used as a working characteristics of completely or partially swirl flows and the parameter tg for swirl flows adjacent to the walls and partially swirl ones along the entire length of the flows (spiral ribbing, wire swirler, etc.).
The parameter tg equals a limit (superficial) tangential of a swirl angle of a flow and is a ratio of superficial tangential of the friction stresses in the tangential and axial directions. The integral parameter Ф* characterizes the ratio of the moment of the amount of movement М to an axial amount of movement К in a random normal to a section which random to a normal to an axial velocity and length of a linear-sized duct :
|
Ф* М / К , |
|
|
(1) |
where |
М 2 R вr2dr , |
K 2 R в |
2rdr, |
(2) |
|
0 |
0 |
|
|
where R is an internal radius of the duct.
For small-scale gas consumption, swirl flowmeters are employed in order to increase the sensitivity of the meters that are fitted with stream swirl devices in the form of axial-tangential
33

Russian Journal of Building Construction and Architecture
swirler as nozzles at the input and output of the measuring tube respectively. The gas consumption is measured using a swirl flowmeter.
A rotating flow that is generated by the input swirl flow meter №1 has a central area that does not move forward. This area has an effect on the linearity of the output characteristics of the device, which influences changes in the physical and technical characteristics of the measured environment (e.g., viscosity, pressure, etc.). In order to mitigate this, the second swirl flow meter №2 is used that swirls the input flow in the reverse direction. This allows the effect of the viscosity on the device to be compensated for and natural pumping losses to be reduced. An effective area of the second swirl flow meter is smaller than that of the first one. Therefore as the external and internal rotating swirls are mixed, the total velocity and rotation direction are formed by the internal rotating with a greater power from the nozzle № 1. The internal swirl reflected from the nozzle № 2 hinders the rotation of the external one. It is obvious that the viscosity of the measured environment changes the velocity of the rotation of both swirls. But as a reduction in the rotation velocity of the internal swirl causes a decrease in the resulting rotating flow, its angular velocity remains constant and does not depend on the viscosity. This scheme is employed with swirl flow meters in the form of jet nozzles where gas comes tangentially to the surface of a device in mutually reverse directions from each nozzle respectively.
The software will be of interest to construction and designing institutions that are involved in the development of new measuring tools.
2. Implementation prospects. The developed software would enable the following:
––determination of the linear velocity of particles in gas in the swirl nuclear;
––determination of the pressure of a swirl outside the swirl nuclear;
––circulation of the velocity for the swirl nuclear in polar coordinates;
––determination of the geometric parameter of the axial and tangential swirl flow meter;
––determination of the growth in the intensity of swirling of the flow;
––graphical visualization of the dependence of the distribution of the axial and tangential velocities on the specified radius of the layer;
––determination of the influence of the weighed phase on changes in the static and dynamic
pressure by visualizing the total changes in the pressure Р.
The software is designed to be employed in communal household services, gas industry, research and everyday practices. Unlike mainstream devices for the same purpose, visualization of changes in the gas pressure in the device allows one to get extra information on small-scale consumption of the gaseous environment and an increase in the sensitivity of the device.
34
Issue № 1 (41), 2019 |
ISSN 2542-0526 |
The software will also come with a restriction as it is not designed for identifying large-scale gas consumption.
The software will be operating managed by the operating system with a graphic interface or another compatible operating system. A processor run PC, memory capacity of no less than 64 МB, hard drive capacity of no less than 10 GB. A graphic printer is necessary for printing out the results.
The developed software can be set up and used on mobile diagnostic and research setups. The software requires no extra software packages as well as special training. One has to be a confident Windows user. Pilot use of the new software will enable them to work with the interface in the period of 5 to 10 minutes.
One of the advantages of the software product is that it can be developed and modified further for the calculation of extra parameters and compatibility with pulse wave record software. This software is used to obtain original scientific results to enhance the involvement of educational institutions into the implementation of the national technological initiative № 13.11847.2018/11.12 “Developing Models for High-Speed Management of Urban Gas Supply Systems Using the Principle of Perturbation Record”.
Conclusions
1.The article looks at the major parameters of the developed software for measuring small-scale natural gas consumption. The software will enable the development and visualization of incoming data in order to identify the linear velocity of gas particles in the swirl nuclear as well as to evaluate the effect of the weighed phase on changes of the static and dynamic pressure.
2.The feature of the developed product is that it requires no special training and software packages. Pilot testing of the new software would enable independent operation of the interface within 5 to 10 minutes. Moreover, if necessary, the software can be further developed and modified for calculating extra parameters and compatibility with pulse wave record software.
3.The obtained results of the research and the software can be applied in energy supply and energy saving systems in gas industry and communal household services. Unlike similar mainstream products, visualization of changes in the pressure of gas movement in the device would allow one to get extra information on small-scale consumption of the gas environment and an increase in the sensitivity of the device.
References
1. Gnezdilova O. A., Kobelev N. S. Matematicheskaya model' sistemy gazosnabzheniya nizkogo davleniya kak energosberegayushchey skhemy operativnogo upravleniya [Mathematical model of low-pressure gas supply sys-
35

Russian Journal of Building Construction and Architecture
tem as energy-saving operational control scheme]. Nauchnyi vestnik Voronezhskogo GASU. Stroitel'stvo i arkhitektura, 2009, no. 3 (15), pp. 32––28.
2.Gnezdilova O. A., Kobelev N. S., Stepanov V. F. Nekotorye polozheniya vikhrevykh protsessov kak osnova dlya rascheta parametrov struezakruchivayushchikh apparatov teplovykh punktov [Some of the provisions of eddies as the basis for calculating the parameters of the jet twisting apparatus for thermal stations]. Izvestiya Kurskogo gosudarstvennogo tekhnicheskogo universiteta, 2007, no. 2 (19), pp. 42––44.
3.Gnezdilova O. A. [The main provisions of the calculation of thermal parameters of the jet twisting devices]. Sbornik materialov XIV rossiiskoi nauchno-tekhnicheskoi konferentsii “Materialy i uprochnyayushchie tekhnologii — 2007” [Proc. of the XIV Russian scientific and technical conference “Materials and strengthening technologies-2007”]. Kursk, 2007, pp. 219––224.
4.Gnezdilova O. A., Kobelev N. S. Osnovy modelirovaniya protsessa upravleniya gorodskimi sistemami gazosnabzheniya srednego i vysokogo davleniya [Basics of modeling the process of management of urban gas supply systems of medium and high pressure]. Nauchnyi vestnik Voronezhskogo GASU. Stroitel'stvo i arkhitektura, 2009, no. 3 (15), pp. 39––46.
5.Gnezdilova O. A. [Improving the accuracy of measurements in the production technology of building materials]. Trudy nauchno-prakticheskoi konferentsii “Sovremennye problemy tekhnicheskogo, estestvennonauchnogo i gumanitarnogo znaniya” [Proc. of the scientific-practical conference “Modern problems of technical, natural science and humanitarian knowledge”]. Stary Oskol, 2005, pp. 260––261.
6.Gnezdilova O. A., Stepanov V. F. [Energy saving management in heating, heating and hot water supply systems]. Trudy XXXIV universitetskoi nauchno-tekhnicheskoi konferentsii studentov i aspirantov v oblasti nauchnykh issledovanii “Molodezh' i XXI vek” [Proc. of the XXXIV University scientific and technical conference of students and postgraduates in the field of research “Youth and XXI century”]. Kursk, 2006, pp. 80––82.
7.Gnezdilova O. A. [Stratification devices as devices that increase the efficiency of coolant control and management]. Sbornik materialov XIV rossiiskoi nauchno-tekhnicheskoi konferentsii “Materialy i uprochnyayushchie tekhnologii — 2007” [Proc. of the XIV Russian scientific and technical conference “Materials and strengthening technologies-2007”]. Kursk, 2007, pp. 225––231.
8.Kobelev N. S., Gnezdilova O. A., Semicheva N. E., Lapin V. A. [The dynamics of the formation of vortex suction compressor, mining machines for quarries]. Sbornik trudov “Vibratsionnye mashiny i tekhnologii” [Proc. of the “Vibrating machines and technologies”]. Kursk, 2008, pp. 689––694.
9.Kobelev N. S., Gnezdilova O. A. [The value of devices for measuring the flow rate, as well as to improve the energy efficiency of coolant transportation]. Trudy mezhdunarodnykh akademicheskikh chtenii “Bezopasnost' stroitel'nogo fonda Rossii. Problemy i resheniya” [Proc. of international academic readings “Safety of the construction Fund of Russia. Problems and solutions”]. Kursk, 2006, pp. 39—42.
10.Ponomarev V. M., Litvinov A. P., eds. Osnovy avtomaticheskogo regulirovaniya i upravleniya [Basics of automatic control]. Moscow, Vysshaya shkola Publ., 1974. 439 p.
11.Panov M. Ya., Kvasov I. S. [Flow distribution models in hydraulic networks based on the variational approach]. Sbornik trudov “Teploobmen v energeticheskikh ustanovkakh i povyshenie effektivnosti ikh raboty”
[Collection of works “Heat Exchange in power plants and improving the efficiency of their work”]. Voronezh, Politekhnicheskii institut Publ., 1991, pp. 101––108.
36
Issue № 1 (41), 2019 |
ISSN 2542-0526 |
12.Panov M. Ya., Kvasov I. S., Kurganov A. M. Universal'naya matematicheskaya model' potokoraspredeleniya gidravlicheskikh setei i usloviya ee sovmestimosti s optimizatsionnymi zadachami [Universal mathematical model of flow distribution of hydraulic networks and conditions of its compatibility with optimization problems]. Izvestiya vuzov. Stroitel'stvo, 1992, no. 11––12, pp. 91––95.
13.Kobelev N. S., Gnezdilova O. A., Zakharov I. S. Schetchik-raskhodomer [Flowmeter]. Patent RF, no. 2003112072/28, 2005.
14.Kobelev N. S., Gnezdilova O. A., Krasnykh P. A., Evdokimov V. D. Izmeritel' raznosti davlenii [Measuring the pressure difference]. Patent RF, no. 2003122444, 2003.
15.Kobelev N. S., Gnezdilova O. A., Shchedrina G. G., Kobelev V. N., Loktionova O. S. Ustroistvo dlya drosselirovaniya gaza [Gas throttling device]. Patent RF, no. 2007102345/22, 2007.
16.Clavelloux N., Mathey R. Analyse mathematique de quelques problemes poses par le gyroscope electrostatique. Doc-Air-Espace, 1966, no. 100, pp. 3––12.
17.Aboubacar M., Phillips T. N., Snigerev B. A. e. a. High-order finite volume methods for viscoelastic flow problems. Physics. V., 2004, no. 199, pp. 16––40.
18.Aboubacar M., Aguayo J. P., Phillips P. M. e. a. Modelling pom-pom type models with high-order finite volume schemes. J. Non-Newtonian Fluid Mech, 2005, vol. 126, pp. 207––220.
19.Atkinson J. L. US pat. No. 3.642.334, cl. 308-10, 15.2. Electrostatic support system, 1972.
37

Russian Journal of Building Construction and Architecture
DOI 10.25987/VSTU.2018.41.1.004
UDC 536.491
N. Yu. Saprykina1, М. Ya. Panov2
INVESTIGATION OF THE INFLUENCE OF THE MODES OF THE OPERATION OF A GEOTHERMAL WELL WITH THE SYSTEMS OF HEAT SUPPLY AND AIR CONDITIONING IN COMBINATION WITH A THERMAL PUMP
ON THE TEMPERATURE OF A SOIL LAYER
Astrakhan State University of Architecture and Civil Engineering Russia, Astrakhan, tel.: +7-927-661-48-60, e-mail: nadin_id@ mail.ru 1Senior lecturer of the Dept. of Engineering Systems and Ecology Voronezh State Technical University
Russia, Voronezh, tel.: (473)271-53-21
2D. Sc. in Engineering, Prof. of the Dept. of Heat and Gas Supply and Oil and Gas Business
Statement of the problem. We discuss the problem of the influence of the operation modes of the heat supply and conditioning systems in combination with the heat pump on the temperature of the soil massif and the development of a methodology for improving the design of these systems taking into account the long-term operation of the heat pump system from a low-potential heat source in a cyclic mode using criteria dependencies for the calculation of well operation parameters.
Results. A description is given of the change in the temperature of the reservoir of the soil massif under the operating conditions of the heat pump in two modes: only for heat supply and alternating heat supply / cooling (air conditioning) for 5 years.
Conclusions. A decrease in the temperature head in the bottomhole part of the geothermal well is revealed, which is used as an unconventional heat source in heat supply and conditioning systems caused by non-stationary thermal loads determined by climatic conditions.
Keywords: heat pump, temperature field, design method, criteria equations.
Introduction. Geothermal heat cannot be commonly used in most settled areas due to small background densities of heat flows. The use of heat pumps for selecting heat of geothermal wells is the solution, but previous experience of operating existing wells shows that technical and economic characteristics of well degrade during operation. Despite that there are still no comprehensible methods in place that would consider these changes [1, 3—5, 8, 10]. Therefore there have been some studies to address long-term prediction of changes in the parameters of geothermal wells operating in heat supply and air conditioning systems and those relying on cyclic changes in these systems.
© Saprykina N. Yu., Panov М. Ya., 2019
38
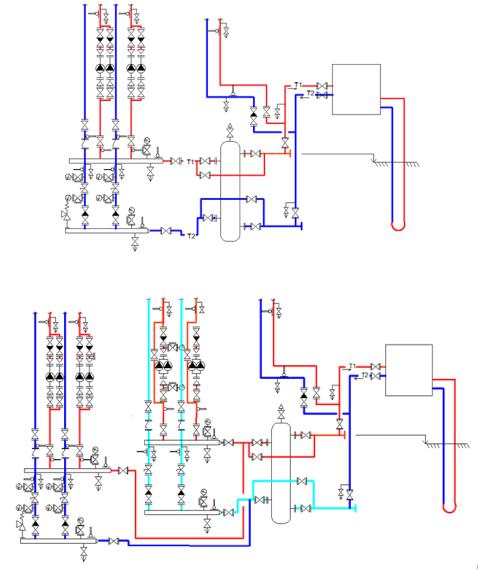
Issue № 1 (41), 2019 |
ISSN 2542-0526 |
1. Modeling a temperature field of soil. A model is suggested for thermal and mass exchange processes in a layer in the influence area of the well including non-stationary heat conductivity [7, 11] and heat transfer with a filtration flow of underground water [13]. Unlike [9, 15], in this paper the problems of calculation modes of a heat-pumping device (HPD) for heat-supply and conditioning systems as well as determining initial and boundary conditions for chosen modes are addressed. For designing a calculation model the following modes (Fig.1) are chosen: a one-flow (only for heat supply or conditioning) and sign-changing (seasonal changes of load accompanied with changes of a flow direction due to switching of a heat pump from the heat supply to conditioning and back).
а)
|
|
|
Heating system (branch № 2) |
|
Heating system (branch № 1) |
|
|
|
|
|
|
HWS system
Heat pump
Ground level marks
Vertical contour (bore)
b)
Heating system (branch № 2)
|
|
|
system |
|
system |
|
|
||||
|
system |
|
№2) |
|
№1) |
|
Heating |
|
rconditioning-Ai |
|
rconditioning-Ai |
|
1) № |
|
(branch |
|
(branch |
|
(branch |
|
|
||
|
|
|
|
|
|
HWS
system
Heat pump
Ground level marks
Vertical contour (bore)
Fig. 1. Principal scheme:
а) heat supply; b) heat supply – conditioning
39