
Учебное пособие 2008
.pdfIssue № 4 (48), 2020 |
ISSN 2542-0526 |
the city of Kashira, Moscow region. The temperature rise in three more cities can be considered inconsiderable. With an increase in the temperature graph, there is a rise in the temperature range spread. The difference in temperature values for the temperature graphs 105/70, 130/70 and 150/70 is 1.05, 2.67 and 3.18 °C, respectively. Due to the inconsiderable difference in the temperature values, it is recommended that the approximate constant values of the average annual temperatures for temperature graphs 105/70, 130/70 and 150/70: 70.8, 75.15 and 79.7 °C are used.
Table 2 shows the results of a comparison of the average annual water temperatures in the supply pipeline with the quality regulation recommended by the latest edition of the Set of Guidelines (СП) “Heating Networks” and obtained as a result of a calculation.
Тable 2
Comparison of the recommended and design average annual temperatures
Design temperature graph |
95/70 |
105/70 |
130/70 |
150/70 |
|
|
|
|
|
|
|
Recommended average annual temperatures in the Set of Guidelines |
65 |
70 |
85 |
90 |
|
(СП) “Heating Networks” |
|||||
|
|
|
|
||
|
|
|
|
|
|
Design average values |
70 |
70.82 |
75.15 |
79.68 |
|
|
|
|
|
|
|
Maximum difference |
5.159 |
1.337 |
11.266 |
12.006 |
|
|
|
|
|
|
|
Minimum difference |
5 |
0.286 |
8.595 |
8.827 |
|
|
|
|
|
|
According to Table. 2, the average calculated values of the average annual water temperatures for the temperature graph 95/70 are 5 °C higher, for 105/70 they differ inconsiderably (0.82 °C), for 130/70 and 150/70 the values are obtained, 9.85 °С and 10.32 °С lower, respectively. The use of values recommended in the Set of Guidelines (СП) for the temperature graph other than 105/70 can lead to considerable errors in the calculations of heating networks and their technical and economic parameters. It is essential to perform similar calculations in each case.
Conclusions. The design values of the average annual water temperatures in the supply pipeline of the heating network for the territory of the Central Federal District may differ considerably from the recommended guidelines. The maximum discrepancy can be 12 °C. The closest are the design values recommended by the guideline with the temperature schedule of 105/70 and as the design temperatures increase, so does the difference.
The use of the values recommended in the Set of Guidelines (СП) can lead to considerable errors in the design of heating networks and calculations of technical and economic characte-
11

Russian Journal of Building Construction and Architecture
ristics. It is suggested that the average annual values are identified by means of a calculation that considers the average monthly temperatures of the coolant depending on the average monthly values of the outside air temperatures for each month of the heating season.
References
1.Badakh V. F., Kuznetsova A. D. Raschet normativnykh poter' tepla cherez izolyatsiyu truboprovodov teplovykh setei [Calculation of standard heat losses through insulation of pipelines of heating networks].
Tekhniko-tekhnologicheskie problemy servisa, 2011, no. 4 (18), pp. 60––72.
2.Bass M. S., Seredkin A. A., Batukhtin S. G [Problems of rationing of heat losses in water heating networks and boiler rooms]. Kulaginskie chteniya: tekhnika i tekhnologii proizvodstvennykh protsessov. XVIII Mezhdunarodnaya nauchno-prakticheskaya konferentsiya [Kulagin readings: technique and technology of production processes. XVIII International Scientific and Practical Conference], 2018, pp. 57––61.
3.Voevodin A. G. Analiz raschetov normativnykh znachenii tekhnologicheskikh poter' pri peredache teplovoi energii po setyam sistem teplosnabzheniya potrebitelei s tsel'yu snizheniya ekspluatatsionnykh zatrat [Analysis of calculations of normative values of technological losses in the transmission of heat energy through the networks of heat supply systems of consumers in order to reduce operating costs]. Transportnye sistemy, 2016, no. 2, pp. 31––41.
4.Gromov N. K., Shubin E. P. Vodyanye teplovye seti: Spravochnoe posobie po proektirovaniyu [Water heating networks: A reference guide for design]. Energoatomizdat, 1988. 376 p.
5.Zhutaeva E. N., Davydova T. E., Dubrovskaya T. N. Optimizatsiya zatrat predpriyatiya (na primere issledovaniya rynka teplosnabzheniya) [Calculation of the outside air temperature at the break point of the temperature graph]. FES: Finansy. Ekonomika, 2017, no. 5, pp. 29––38.
6.Kitaev D. N. Raschet temperatury naruzhnogo vozdukha v tochke izloma temperaturnogo grafika [Modern heating devices and their indicators]. Novosti teplosnabzheniya, 2012, no. 10 (146), pp. 46––48.
7.Kitaev D. N. Sovremennye otopitel'nye pribory i ikh pokazateli [On the influence of the coolant temperature on the technical and economic indicators of the projected heating networks]. Santekhnika, otoplenie, konditsionirovanie, energosberezhenie, 2014, no. 1, pp. 48––49.
8.Kononova M. S. O vliyanii temperatury teplonositelya na tekhniko-ekonomicheskie pokazateli proektiruemykh teplovykh setei [On the influence of the coolant temperature on the technical and economic indicators of the projected heating networks]. Izvestiya vuzov. Stroitel'stvo, 2012, no. 10, pp. 67––73.
9.Nurov M. Sh., Gordin S. A. [Optimization of the heat supply scheme according to the criterion of minimum losses when transporting energy to the consumer]. Molodezh' i nauka: aktual'nye problemy fundamental'nykh i prikladnykh issledovanii. Materialy II vserossiiskoi natsional'noi nauchnoi konferentsii studentov, aspirantov i molodykh uchenykh [Youth and Science: Actual Problems of Fundamental and Applied Research. Materials of the II All-Russian National Scientific Conference of Students, Postgraduates and Young Scientists], 2019. Pp. 392––395.
10.Panferov V. I., Anisimova E. Yu., Panferov S. V. Effektivnye energosberegayushchie resheniya pri teplosnabzhenii zdanii [Effective energy saving solutions for heat supply of buildings]. Vestnik YuzhnoUral'skogo gosudarstvennogo universiteta. Seriya: Stroitel'stvo i arkhitektura, 2015, vol. 15, no. 4, pp. 40––48.
12
Issue № 4 (48), 2020 |
ISSN 2542-0526 |
11.Panferov V. I., Panferov S. V. Ob osobennostyakh vyvoda uravnenii regulirovaniya sistem tsentralizovannogo teplosnabzheniya [On the features of the derivation of the equations of regulation of centralized heating systems]. Vestnik Yuzhno-Ural'skogo gosudarstvennogo universiteta. Seriya: Komp'yuternye tekhnologii, upravlenie, radioelektronika, 2016, vol. 16, no. 1, pp. 21––30.
12.Kitaev D. N., Novakovski P., Sazonov E. V. e. a. Prakticheskoe primenenie energosberegayushchikh tekhnologii [Practical application of energy-saving technologies]. Tambov, Izd-vo Pershina R. V., 2014. 193 p.
13.Rafal'skaya T. A. Nizkotemperaturnye rezhimy raboty teplovykh setei pri kachestvenno-kolichestvennom regulirovanii [Low-temperature operating modes of heating networks with qualitative and quantitative regulation]. Novaya nauka: Teoreticheskii i prakticheskii vzglyad, 2016, no. 10 (2), pp. 141––143.
14.Sister V. G., Yamchuk A. I., Polivoda F. A. Matematicheskaya model' teplovoi seti i razrabotka rekomendatsii po raschetu kpd dlya proizvol'nogo temperaturnogo grafika [Mathematical model of a heating network and development of recommendations for calculating efficiency for an arbitrary temperature graph]. Izvestiya Moskovskogo gosudarstvennogo tekhnicheskogo universiteta MAMI, 2012, vol. 4, no. 2 (14), pp. 318––324.
15.Smorodova O. V., Kitaev S. V., Useev N. F. Sravnitel'nyi analiz metodov regulirovaniya teplosnabzheniya [Comparative analysis of heat supply regulation methods]. Norwegian Journal of Development of the International Science, 2018, no. 17-1, pp. 54––58.
16.Khismatullin Sh. Kh., Ucharov U. B., Kashitsyn V. P., Chulkova S. A. O temperaturnom grafike raboty teplovykh setei g. Kazani [On the temperature schedule of the heat networks in Kazan]. Energetika Tatarstana, 2011, no. 2 (22), pp. 39––43.
17.Sharapov V. I., Rotov P. V. Regulirovanie nagruzki sistem teplosnabzheniya [Regulation of the load of heat supply systems]. Moscow, Novosti teplosnabzheniya Publ., 2007. 164 p.
18.Akhmetova I., Chichirova N., Derevianko O. Revisiting heat losses calculation at district heating network.
International Journal of Civil Engineering and Technology, 2017, vol. 8, no. 12, pp. 694––702.
19.Kitaev D., Bugaevsky D., Makarov A. Modeling the work of a steam-water injector in a heat supply system. E3S Web of Conferences. Key Trends in Transportation Innovation, KTTI 2019, 2020. P. 06037.
20.Martynenko G. N., Kitaev D. N., Sedaev A. A. Prospects for the development of the gas supply system of the city district of voronezh for the period till 2035. Russian Journal of Building Construction and Architecture, 2018, no. 4 (40), pp. 26––39.
21.Melkumov V. N., Kuznetsov S. N., Sklyarov K. A., Gorskikh A. A. Reliability monitoring of heat supply networks. Scientific Herald of the Voronezh State University of Architecture and Civil Engineering. Construction and Architecture, 2011, no. 1 (9), pp. 42––49.
22.Melkumov V. N., Sklyarov K. A., Tulskaya S. G., Chuikina A. A. Criteria of optimality and condition of the comparison of design solutions of systems of heat supply. Russian Journal of Building Construction and Architecture, 2018, no. 1 (37), pp. 18––28.
23.Shan X., Wang P., Lu W. The reliability and availability evaluation of repairable district heating networks under changeable external conditions. Applied Energy, 2017, vol. 203, pp. 686––695.
24.Sharapov V. I., Rotov P. V., Orlov M. E. The improvement technologies of the thermal load regulation for cogeneration systems in urban areas. Transactions of Academenergo, 2010, no. 4, pp. 70––83.
13
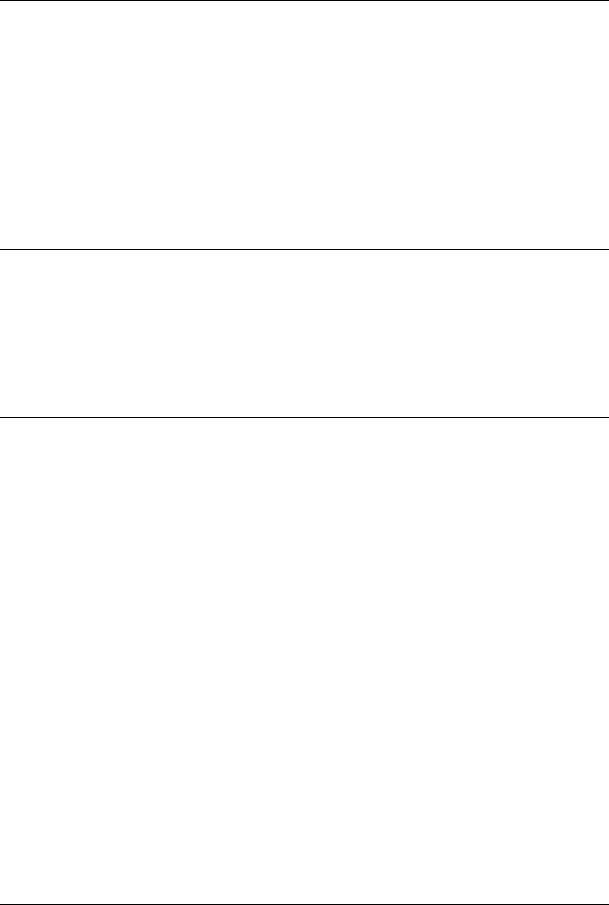
Russian Journal of Building Construction and Architecture
DOI10.36622/VSTU.2020.48.4.002 UDC697.91
V. N. Melkumov1, G. A. Kuznetsova2, A. V. Panin3, M. Ya. Panov4
USING AIR EXCHANGE TO REDUCE THE PROBABILITY
OF SPREADING CORONAVIRUS INFECTION
Voronezh State Technical University1, 2, 3, 4
Russia, Voronezh
1D. Sc. in Engineering, Prof. of the Dept. of Heat and Gas Supply and Oil and Gas Business, tel.: (473) 271-53-21, e-mail: teplosnab_kaf@vgasu.vrn.ru
2PhD in Engineering, Assoc. Prof. of the Dept. of Heat and Gas Supply and Oil and Gas Business, tel.: (473) 271-53-21, e-mail: ga_kuzn@mail.ru
3PhD in Engineering, Assoc. Prof. of the Dept. of Heat and Gas Supply and Oil and Gas Business, tel.: (473) 271-53-21, e-mail: panin@vgasu.vrn.ru
4PhD in Engineering, Assoc. Prof.of the Dept. of Heat and Gas Supply and Oil and Gas Business, tel.: (473) 271-53-21
Statement of the problem. Ventilation processes have a significant impact on the spread of airborne infections. It is necessary to use air exchange to reduce the likelihood of spreading such infections.
Mathematical model. Using the Wells - Riley model of airborne transmission of infectious diseases, a mathematical model has been developed for the spread of coronavirus infection in a medical institution, consisting of a group of communicating rooms in which both healthy and infected people are constantly located and moved. The mathematical model makes it possible to take into account the movement of people around the premises and the settling of quanta of the generation of infection by a sick person when air moves.
Results. The general solution of the mathematical model is obtained, which allows calculating the concentration of quanta of generation of infection in the premises during the operation of a medical institution.
Conclusions. The developed mathematical model of a medical institution allows a deeper understanding of the possibilities of the spread of coronavirus infection and taking these risks into account when designing medical institutions.
Keywords: ventilation, hospitals, coronavirus.
Introduction. A lot of studies indicate that ventilation affects the likelihood of contracting airborne infections such as influenza and rhinovirus infections [18]. The latter include the coronavirus (COVID-19), the pandemic which is currently gaining momentum.
© Melkumov V. N., Kuznetsova G. A., Panin A. V., Panov M. Ya., 2020
14
Issue № 4 (48), 2020 |
ISSN 2542-0526 |
The process of transmission of infection by airborne droplets has multiple aspects to it. This paper discusses the use of air exchange created by ventilation systems to reduce the likelihood of the spread of infections transmitted by airborne droplets.
The concept of the spread of airborne infections was first described by Wells [21] and then by Riley [14, 15, 20]. The Wells-Riley equation [20] was employed to assess the effect of ventilation, filtration, and other physical processes on transmission through droplet nuclei [7, 9, 11, 12]. The detection of pathogenic microorganisms in the air of the premises of medical institutions in the absence of patients indicates a possible indirect connection between the processes of room-to-room air flow and the transmission of diseases [13, 16, 18, 19].
The objective of this work is to assess the effect of general ventilation on the spread of coronavirus infection in a medical institution.
1. Mathematical model of the transmission of the coronavirus infection by means of general ventilation. For individuals in the same room, the probability of contracting an infectious disease transmitted by means of airborne droplets is estimated by the Wells-Riley dependence [20]:
|
D |
1 e |
Ipqt |
|
|
P |
Q |
, |
(1) |
||
|
S |
|
|
|
|
where D is the number of cases; S is the total number of people in the room; I is the number of sick people in the room; p is the human respiration rate, m³/sec; q is the rate of quantum generation of infection by a sick person (quantum/sec); t is the total time spent in the room, sec; Q is the amount of clean air entering the room per unit of time, m³/sec.
The rate of generation of quanta of infection by a sick person was introduced by Wells [21]. He argued that not all inhaled core droplets would lead to infection and defined the infection quantum as the number of infectious droplet nuclei required for infection. Equation (1) is based on a Poisson distribution and assumes that only one new infection is likely to occur in a fairly short time period.
Based on the Wells-Riley dependence, the number of cases can be expressed:
|
|
|
|
Ipqt |
|
|
|
|
|
|
D S 1 e |
|
Q |
. |
|
|
|
|
|||
|
|
|
|
|
|
|
|
|
|
|
|
|
|
|
|
|
|
|
|
|
|
Or summing for all the facilities in a building |
|
|
|
|
|
|
|
|
|
|
n |
|
n |
|
|
|
|
Ii pqt |
|
||
i |
|
|
|
i |
|
|
|
|
|
|
D |
|
|
S |
1 |
e Qi |
|
, |
|||
i 1 |
|
i 1 |
|
|
|
|
|
|
|
|
where n is the total number of rooms in a medical institution.
(2)
(3)
15

Russian Journal of Building Construction and Architecture
Let us consider the problem of designing effective ventilation of a medical institution comprised of a group of adjoining rooms where both healthy and infected people constantly reside and move around.
Indoor air quality is predominantly defined by air exchange. General exchange ventilation of premises can be mixing ventilation (MV), displacement ventilation (DV) or a combination of both [1, 3]. While mixing ventilation is employed, turbulent air flows are intensively mixed, and highly dispersed particles are relatively evenly distributed along the room [4, 5, 17]. While displacement ventilation is employed, laminar air flows are designed which enables highly dispersed particles to be directed through the room. Displacement ventilation generates a more efficient air exchange in the room, but its use increases capital and operating costs considerably [6, 8, 10, 22].
Let us compose the equation of air exchange of a medical institutio, consisting of a group of communicating rooms with MV ventilation type. The number of quanta of infection generated by a sick person coming from adjoining rooms will be:
|
|
|
|
|
|
|
|
cj Lji ji dt , |
|
(4) |
where |
|
|
is the concentration of quanta of the generation of infection by a sick person in the |
|||||||
j-th room, |
quantum m-2; |
|
is the amount of air supplied from the j-th room to the i-th room, |
|||||||
3 |
-1 |
|
|
|
|
coefficient of settling of quanta of infection generated by a sick person |
||||
m sec |
|
; |
|
|
is the |
|||||
|
|
|
|
|
|
|
|
|||
when air moves from the j-th room to the i-th room; dt is a time increment, sec. |
|
|
||||||||
|
|
|
|
|
|
|
|
|
|
|
The number of quanta of infection generated from patients entering the room will be: |
|
|
||||||||
|
|
|
|
|
|
|
|
Ii pq dt , |
|
(5) |
where |
|
|
is the number of patients in the i-th room. |
|
|
|||||
The amount |
of quanta of infection generated by a sick person into adjoining rooms and into |
|||||||||
the surrounding air will be: |
|
|
|
|||||||
|
|
|
|
|
|
|
|
ci Lij dt ci Li0dt , |
|
(6) |
where |
|
|
is the amount of air flowing from the i-th room into the j-th room, m3 sec-1; |
id |
||||||
the |
|
|
|
|
|
|
|
|
amount of air flowing from the i-th room into the environment, m3 sec-1.
Then we obtain the differential equation of the material balance for the quanta of infection generation by a sick person:
n |
n |
|
cj Lji ji dt Ii pqdt ci Lij dt ci Li0dt Vi dci , |
(7) |
|
j 1 |
j 1 |
|
j i |
j i |
|
where is the area of the i-th room, m3.
16
Issue № 4 (48), 2020 |
ISSN 2542-0526 |
By means of transformation we get
cj Lji ji Ii pq ci Lij ci Li0 Vi |
dci . |
(8) |
|
n |
n |
|
|
j 1 |
j 1 |
dt |
|
j i |
j i |
|
|
For the entire medical institution consisting of a group of communicating rooms, we obtain a system of ordinary differential equations:
n |
n |
|
|
dc |
|
|
||
cj Lj1 j1 |
I1pq c1L1j c1L10 V1 |
|
1 |
|
|
|
||
|
|
|
|
|
|
|
|
|
j 1 |
j 1 |
|
dt |
|
|
|||
j 1 |
j 1 |
|
|
|
|
|
|
|
n |
|
n |
|
|
dc2 |
|
|
|
cj Lj 2 j 2 I2 pq c2L2 j c2L20 |
V2 |
|
|
|||||
j 1 |
j |
1 |
|
|
dt |
. |
(9) |
|
j 2 |
j |
2 |
|
|
|
|
||
|
|
|
|
|
|
|
|
|
|
|
|
||||||
|
|
|
|
|
|
|
|
|
n |
n |
|
|
dcn |
|
|
|
|
cj Ljn jn In pq cn Lnj cn Ln0 |
Vn |
|
|
|||||
dt |
|
|
||||||
|
|
|
|
|
|
|||
j 1 |
j |
1 |
|
|
|
|
|
|
j n |
j n |
|
|
|
|
|
|
Transforming a system of ordinary differential equations (9), we get
dc |
n |
Lj1 j1 |
|
|
|
|
n L1j |
|
|
|
|
L |
|
|
|
I pq |
|
|||||||||||||||||||||
|
1 |
cj |
|
|
|
|
c1 |
|
|
|
|
|
|
|
|
|
|
|
10 |
|
|
|
|
1 |
|
|
|
|
|
|
|
|||||||
|
|
V |
|
V |
|
V |
|
|
V |
|
|
|
|
|
||||||||||||||||||||||||
dt |
j 1 |
|
|
|
|
|
j 1 |
|
|
|
|
|
|
|
|
|
|
|
|
|
||||||||||||||||||
|
|
|
|
|
1 |
|
|
|
|
|
|
|
|
1 |
|
|
|
|
|
|
|
1 |
|
|
|
|
|
|
1 |
|
|
|
|
|
||||
|
j 1 |
|
|
|
|
|
|
j 1 |
|
|
|
|
|
|
|
|
|
|
|
|
|
|
|
|
|
|
|
|
|
|
|
|
|
|||||
|
|
|
|
|
|
|
|
|
|
|
|
|
|
|
|
|
|
|
|
|
|
|
|
|
|
|
|
|
|
|
|
|
|
|
|
|
|
|
|
dc |
n |
|
L |
|
|
|
|
n |
|
|
L |
|
|
|
|
|
|
|
L |
|
|
|
|
|
I |
|
pq |
|
|||||||||
|
cj |
|
|
j 2 j 2 |
|
c2 |
|
|
|
2 j |
|
|
|
|
|
|
|
2 |
|
|||||||||||||||||||
|
2 |
|
|
|
|
|
|
|
|
|
|
|
20 |
|
|
|
|
|
|
|
(10) |
|||||||||||||||||
|
|
V |
|
|
V |
|
|
V |
|
|
|
V |
||||||||||||||||||||||||||
|
dt |
j 1 |
|
|
|
|
|
j 1 |
|
|
|
|
|
|
|
|
|
|
|
|
|
|
|
|||||||||||||||
|
|
|
|
|
2 |
|
|
|
|
|
|
|
|
2 |
|
|
|
|
|
|
|
|
2 |
|
|
|
|
|
|
|
|
2 |
|
|
|
|||
|
j 2 |
|
|
|
|
|
|
|
j 2 |
|
|
|
|
|
|
|
|
|
|
|
|
|
|
|
|
|
|
|
|
|
|
|
|
|
||||
|
|
|
|
|
|
|
|
|
|
|
|
|
|
|
|
|
|
|
|
|
|
|
|
|
|
|
|
|
|
|
|
|
|
|
|
|
|
|
|
|
|||||||||||||||||||||||||||||||||||||
|
|
|
|
|
|
|
|
|
|
|
|
|
|
|
|
|
|
|
|
|
|
|
|
|
|
|
|
|
|
|
|
|
|
|
|
|
|
|
dc |
n |
|
Ljn jn |
|
|
n |
|
Lnj |
|
|
|
|
|
|
L |
0 |
|
|
|
I |
n |
pq |
|
|||||||||||||||
|
n |
cj |
|
|
|
|
|
|
|
|
|
|
|
|
|
|
|
|
|
|
n |
|
|
|
|
|
|
|
|
|
|
|
||||||
|
|
|
|
|
|
|
cn |
|
|
|
|
|
|
|
|
|
|
|
|
|
|
|
|
|
|
|
|
|
|
|||||||||
dt |
|
|
Vn |
|
Vn |
|
|
|
|
Vn |
|
|
Vn |
|
||||||||||||||||||||||||
j 1 |
|
|
|
|
j 1 |
|
|
|
|
|
|
|
|
|
|
|
|
|
||||||||||||||||||||
|
|
|
|
|
|
|
|
|
|
|
|
|
|
|
|
|
|
|
|
|
|
|
|
|
|
|
|
|
|
|
|
|
|
|
|
|
|
|
|
|
j n |
|
|
|
|
|
|
j n |
|
|
|
|
|
|
|
|
|
|
|
|
|
|
|
|
|
|
|
|
|
|
|
|
|
||||
|
|
|
|
|
|
|
|
|
|
|
|
|
|
|
|
|
|
|
|
|
|
|
|
|
|
|
|
|
|
|
|
|
|
|
|
The initial condition for the system of ordinary differential equations (10) is the concentration of quanta of infection generation by a sick person in the premises at the initial moment of time.
By means of transformion of the system of ordinary differential equations (10), we get
dc1 |
m c |
m |
c m |
c |
|
I1 pq |
|
|
|
|
|||||
|
V |
|
|||||||||||||
dt |
12 |
2 |
13 |
3 |
1n |
|
n |
|
|
|
|
||||
|
|
|
|
|
|
|
|
|
|
1 |
|
|
|
|
|
dc2 |
m |
c m |
c m |
|
c |
|
I2 pq |
|
|
|
|||||
|
|
|
|
||||||||||||
|
21 1 |
23 3 |
2n |
n |
|
|
|
V2 . |
(11) |
||||||
dt |
|
|
|
|
|
|
|
|
|
|
|||||
|
|
|
|
|
|
|
|
|
|
|
|
|
|
|
|
|
|
||||||||||||||
|
|
|
|
|
|
|
|
|
|
|
|
|
|
|
|
dcn |
m c |
m |
c |
m c |
|
In pq |
|
|
|||||||
|
V |
|
|||||||||||||
dt |
n1 2 |
n2 2 |
n3 n |
|
|
|
|
|
|
||||||
|
|
|
|
|
|
|
|
|
|
|
n |
|
17

Russian Journal of Building Construction and Architecture
Let us denote:
|
0 |
m12 |
|
m |
0 |
|
|
M |
21 |
|
|
|
|
||
|
|
mn2 |
|
mn1 |
m1n m2n
0
|
|
|
|
|
|
|
|
|
dc |
|
|
|
|
|
|
c1 |
|
|
|
|
|
|
1 |
|
|
|
|
|
|
|
|
|
|
|
dt |
|
|
|
|
|
|
|
|
|
|
|
|
dc2 |
|
|
|
|
, |
C |
c2 |
|
, |
C |
|
|
|
dt |
|
, |
|
|
|
|
|
||||||||
|
|
|
|
|
|
|
|
|
|
|
|
|
|
|
|
cn |
|
|
|
|
|
dc |
|
|
|
|
|
|
|
|
|
|
|
|
|
n |
|
|
|
|
|
|
|
|
|
|
|
|
dt |
|
|
|
|
I1 pq |
|
|||||
|
|
|
|
|
|
|
||
|
V |
|
||||||
|
|
1 |
|
|
|
|
||
|
|
I2 pq |
|
|||||
I |
|
|
|
|
|
. |
(12) |
|
|
V |
|
||||||
|
|
2 |
|
|
|
|
||
|
|
|
|
|
|
|
||
|
|
|
|
|
|
|
||
|
|
In pq |
|
|||||
|
|
|
V |
|
|
|
|
|
|
|
n |
|
|
|
|
2. General solution of the equations of the mathematical model of the transmission of the coronavirus infection by means of general ventilation. Matrix M characterizes the movement of infection generation quanta in the air of the premises of a medical institution considering their settling. I characterizes the release of quanta of infection generation in the facili-
ties of a medical institution depending on the number of patients. |
|
Let us write the system of ordinary differential equations (12) in the following form: |
|
C MC I . |
(13) |
With the initial condition in the form of initial concentrations of quanta of infection generation in the j-th room, quantum m-2:
|
|
c10 |
|
|
|
|
|
c |
|
(14) |
|
|
C |
20 |
. |
||
|
0 |
|
|
|
|
|
|
|
|
|
|
|
|
cn0 |
|
|
|
Generally, the solution to the system of ordinary differential equations (13) can be represented as |
|||||
C t |
e t M Id e t t0 M C0 . |
(15) |
|||
t0 |
|
|
|
|
|
The opening of doors between rooms is modeled by a sequence of matrices M1, M2, M1 ... The movement of sick people releasing quanta of infection generation is modeled by means of a sequence of vectors:
|
|
|
I11 pq |
|
||||
|
|
|
|
|
|
|
||
|
|
V |
|
|||||
|
|
|
1 |
|
|
|
||
|
|
|
I21 pq |
|
||||
I |
|
|
|
|
|
|
|
, |
|
|
|
V |
|
||||
1 |
|
|
2 |
|
|
|
||
|
|
|
|
|
|
|
||
|
|
|
|
|
|
|
||
|
|
|
In1 pq |
|
||||
|
|
|
|
V |
|
|
|
|
|
|
|
n |
|
|
|
|
|
|
|
I12 pq |
|
|||||
|
|
|
|
|
|
|
|
|
||
|
|
|
V |
|
||||||
|
|
|
|
1 |
|
|
|
|
||
|
|
|
|
I22 pq |
|
|||||
I |
|
|
|
|
|
|
|
|
, |
|
2 |
|
|
V |
|
||||||
|
|
|
2 |
|
|
|
|
|||
|
|
|
|
|
|
|
|
|
||
|
|
|
|
|
|
|
|
|
||
|
|
|
|
In2 pq |
|
|||||
|
|
|
|
|
V |
|
|
|
|
|
|
|
|
|
n |
|
|
|
|
|
|
|
|
I13 pq |
|
|
|||||
|
|
|
|
|
|
|
|
|
|
||
|
|
|
V |
|
|
||||||
|
|
|
|
1 |
|
|
|
|
|
||
|
|
|
|
I23 pq |
|
|
|||||
I |
|
|
|
|
|
|
|
|
… . |
(16) |
|
3 |
|
|
V |
|
|||||||
|
|
|
2 |
|
|
|
|
|
|||
|
|
|
|
|
|
|
|
|
|
||
|
|
|
|
|
|
|
|
|
|
||
|
|
|
|
In3 pq |
|
|
|||||
|
|
|
|
|
V |
|
|
|
|
|
|
|
|
|
|
n |
|
|
|
|
|
18
Issue № 4 (48), 2020 |
ISSN 2542-0526 |
Using the developed mathematical model, it is possible to calculate the concentration of infection generation quanta in the premises of a medical institution for any air exchange with variable air flow between rooms, which makes it for the risks of infection in healthy people to be assessed.
Conclusions. Mass exchange processes caused by the operation of general exchange ventilation systems have a significant impact on the spread of infections transmitted by airborne droplets. The room-to-room air movement must be controlled to reduce the risk of spreading such infections.
Based on the Wells-Riley model of airborne transmission of infectious diseases and the differential equation of air exchange, a mathematical model of the spread of the coronavirus infection in a medical institution comprised of a group of adjoining rooms with air overflow has been developed. Both healthy and infected people can be in the facilities permanently or temporarily. The mathematical model allows for the possibility of settling of quanta of infection generation by a sick person in the facilities.
Based on the resulting general solution of the equations of the mathematical model, the concentration of quanta of the generation of infection in the premises during the operation of a medical institution can be calculated.
The mathematical model provides a deeper insight into the possibilities of the spread of the coronavirus infection and considering these risks in the process of designing medical institutions.
References
1.Mel'kumov V. N., Kuznetsov S. N., Gulak V. V. Modelirovanie zadymlennosti pomeshchenii slozhnoi konfiguratsii v nachal'noi stadii pozhara [Simulation of the smoke content of rooms of complex configuration at the initial stage of a fire]. Nauchnyi vestnik VGASU. Stroitel'stvo i arkhitektura, 2010, no. 3 (19), pp. 131––139.
2.Mel'kumov V. N., Kuznetsov S. N., Pavlyukov S. P., Cheremisin A. V. Nestatsionarnoe pole kontsentratsii prirodnogo gaza v skvazhine pri ego utechke iz podzemnogo gazoprovoda [Unsteady field of natural gas concentrations in a well when it leaks from an underground gas pipeline]. Privolzhskii nauchnyi zhurnal, 2008, no. 4 (8), pp. 98––103.
3.Mel'kumov V. N., Kuznetsov S. N., Sklyarov K. A., Cheremisin A. V. Nestatsionarnye protsessy formirovaniya sistemami ventilyatsii vozdushnykh potokov v pomeshcheniyakh [Non-stationary processes of formation by ventilation systems of air flows in rooms]. Izvestiya OrelGTU. Ser. Stroitel'stvo. Transport, 2007, no. 3-15 (537), pp. 36––39.
4.Mel'kumov V. N., Kuznetsov S. N., Lachugin I. G., Sviridov A. A. O metodike rascheta kontsentratsii prirodnogo gaza pri nalichii utechki iz podzemnogo gazoprovoda [On the methodology for calculating natural gas concentrations in the presence of a leak from an underground gas pipeline]. Vestnik VGTU, Ser. Energetika, 2001, vol. 7.1, pp. 72––75.
19

Russian Journal of Building Construction and Architecture
5.Polosin I. I., Derepasov A. V. Modelirovanie ventilyatsionnykh protsessov v proizvodstvennykh pomeshcheniyakh s pro-emami v mezhduetazhnykh perekrytiyakh [Simulation of ventilation processes in industrial premi-ses with openings in the interfloor floors]. Nauchnyi vestnik VGASU. Stroitel'stvo i arkhitektura, 2011, no. 2 (22), pp. 43––51.
6.Polosin I. I., Lobanov D. V. Raschet trebuemogo vozdukhoobmena v ofisnom pomeshchenii pri organizatsii perso-nal'noi sistemy ventilyatsii [Calculation of the required air exchange in the office space when organizing a personal ventilation system]. Privolzhskii nauchnyi zhurnal, 2014, no. 1 (29), pp. 56––60.
7.Allen K. D., Green H. T. Hospital outbreak of multi-resistant Acinetobacter anitratus: an airborne mode of spread. Journal of Hospital Infection, 1987, no. 9, pp. 110––119.
8.ASHRAE. HVAC Design Manual for Hospitals and Clinics. 2nd ed. Atlanta: American Society of Heating, Refrigerating and Air-Conditioning Engineers, 2013.
9.Bartrand T. A., Weir M. H., Haas C. N. Doseresponse models for inhalation of Bacillus anthracis spores: interspecies comparisons. Risk Anal, 2008, no. 28, pp. 1115––1124.
10.Beggs C. B., Sleigh P. A. A quantitative method for evaluating the germicidal effect of upper room UV fields. Journal of Aerosol Science, 2002, no. 33, pp. 1681––1699.
11.Chen Q. Ventilation performance prediction for buildings: a method overview and recent applications. Build. Environ, 2009, no. 44, pp. 848––858.
12.Escombe A. R. Upper-room ultraviolet light and negative air ionization to prevent tuberculosis transmission. PLoS Med, 2009, no. 6, pp. 1––12.
13.Fennelly K. P., Martyny J. W., Fulton K. E., Orme I. M., Cave D. M., Heifets L. B. Coughgenerated aerosols of Mycobacterium tuberculosis: a new method to study infectiousness. Am J Respir Crit Care Med, 2004, no. 1, pp. 1––5.
14.Fennelly K. P., Nardell E. A. The relative efficacy of respirators and room ventilation in preventing occupational tuberculosis. Infect Control Hosp Epidemiol, 1998, no. 19 (10), pp. 754––759.
15.Jones R. M., Masago Y., Bartrand T. A., Haas C. N., Nicas M., Rose J. B. Characterizing the risk of infection from Mycobacterium tuberculosis in commercial passenger aircraft using quantitative microbial risk assessment. Risk Anal, 2009, no. 29, pp. 355––365.
16.Kumari D. N. P. Ventilation grilles as a potential source of methicillin-resistant Staphylococcus aureus causing an outbreak in an orthopaedic ward at a district general hospital. Journal of Hospital Infection, 1998, no. 39, pp. 127––133.
17.Noakes C. J., Sleigh P. A. Mathematical models for assessing the role of airflow on the risk of airborne infection in hospital wards. Soc Interface, 2009, no. 6, pp. 791––800.
18.Qian H., Li Y. G., Nielsen P. V., Huang X. H. Spatial distribution of infection risk of SARS transmission in a hospital ward. Build. Environ, 2009, no. 44, pp. 1651––1658.
19.Riley E. C., Murphy G., Riley R. L. Airborne spread of measles in a suburban elementary school. American Journal of Epidemiology, 1978, no. 107, pp. 421––432.
20.Wells W. F. Airborne Contagion and Air Hygiene: An Ecological Study of Droplet Infections. Harvard University Press, 1955. 423 p.
21.Xie X., Li Y., Chwang A. T., Ho P. L., Seto W. H. How far droplets can move in indoor environmentsrevisiting the Wells evaporation-falling curve. Indoor Air, 2007, no. 17 (3), pp. 211––25.
20